Author Affiliations
1Shanghai Advanced Research Institute, Chinese Academy of Sciences, Shanghai 201210, China2Center for Terahertz Waves and College of Precision Instrument and Optoelectronics Engineering, Key Laboratory of Opto-electronics Information and Technical Science, Ministry of Education, Tianjin University, Tianjin 300072, China3University of Chinese Academy of Sciences, Beijing 100049, China4ShanghaiTech University, Shanghai 201210, China5e-mail: zhangyz@sari.ac.cn6e-mail: yantm@sari.ac.cn7e-mail: jiangyh@sari.ac.cnshow less
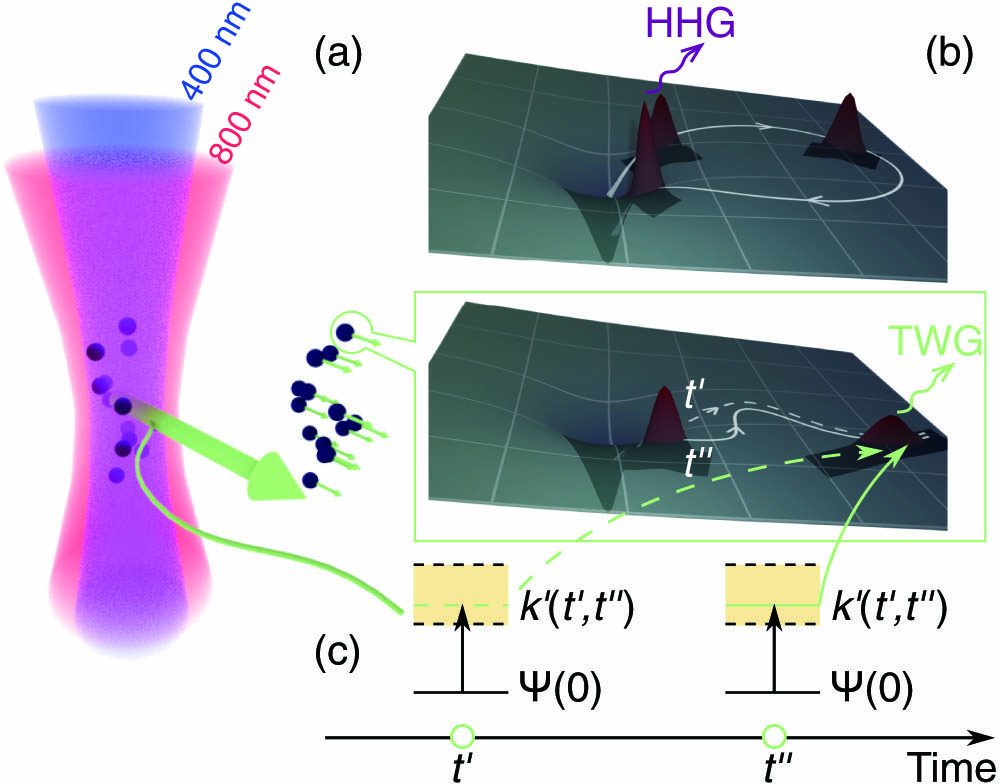
Fig. 1. Illustrative description of the TWG in dual-color fields and the mechanism. In panel (a), the dual-color laser ionizes the gas-phase medium and accelerates the electrons. According to the photocurrent model from the plasma perspective, the TWG originates from the rapidly varying macroscopic residual current as indicated by the giant arrow. However, the strong-field theory provides an alternative explanation from the microscopic aspect that each atom behaves as an individual THz emitter. As illustrated by the swarm of particles, each with an arrow indicates the THz radiation from a single atom. Panel (b) presents the radiation mechanisms under the strong-field approximation. After the photoelectron is released by the external light fields from the distorted atomic potential, it may recollide with its parent core, yielding the HHG; or within the continuum wave packet, the transition between continuum states of similar energies leads to the TWG. According to the derived SFA-CC, the involved continuum states are via different quantum paths as indicated by the white solid and dashed lines on the potential surface in (b). Panel (c) shows the origin of the two paths. The photoelectrons of initial state |Ψ(0)⟩ are released at different ionization times t′ and t′′ but arrive at the same intermediate momentum state k′(t′,t′′). The coherence between the continuum states yields the TWG.
Fig. 2. Comparison of PP distributions, STHz,s(τ,θ) (upper row) and STHz,p(τ,θ) (lower row), respectively, for the s and p components of ETHz(t) obtained from (a) experiment and (b)–(d) theoretical models of the (b) SFA-CB, (c) SFA-CC, and (d) SPC.
Fig. 3. w(t′;t) of the SFA-CC and the comparison with w(t′) of the SPC when θ=0° and τ=0.33 fs. In collinear dual-color laser fields, the SFA-CC derived w1(t′;t) and w2(t′;t) are shown in (a) and (b), respectively, with their detailed zoom-in around t′=0 in insets (c) and (d). The total contribution, w(t′;t)=w1(t′;t)+w2(t′;t), is presented in inset (e), showing that w2(t′;t) almost contributes at t′=t only. In (f), w(t′;t→∞) of the SFA-CC (solid line) is compared with w(t′) of the SPC (dashed line), showing the correspondence between the SFA-based quantum model and the semi-classical PC model.
Fig. 4. Experimental setup. BS, beam splitter; CH, chopper; β-BBO, beta barium borate; DWP, dual-wavelength plate; PM, parabolic mirror; QWP, quarter-wave plate; GLP, Glan-laser polarizer; WP, Wollaston polarizer; BD, balanced detector.
Fig. 5. Definition of observables. The 800 nm (ω) and 400 nm (2ω) beams collinearly propagate. The 2ω beam is always s polarized. The relative time delay τ and polarization angle θ are controlled in the measurement. Both the s- and p-polarized terahertz waves are detected.
Fig. 6. Distribution of the THG along s-polarization I3rd(τ,θ) obtained from (a) the measurement, (b) d¨(1)(t) of SFA-CB, (c) d¨(2)(t) of SFA-CC, and (d) SPC.
Fig. 7. (a) STHz,s(τ,θ) and (b) STHz,p(τ,θ) evaluated from the PC model.
Fig. 8. (a) STHz,s(τ=0.33 fs,θ) and (b) STHz,s(τ,θ=90°) of the experiment (red star), PC (blue dashed), and SPC (magenta dotted) models.