Yang DONG, Jing-Yu GUO, He-Xin WANG, Zhan-Liang WANG, Zhi-Gang LU, Hua-Rong GONG, Zhao-Yun DUAN, Yu-Bin GONG, and Shao-Meng WANG*
Abstract
A dual-beam rectangular ring-bar (DBRRB) slow wave structure (SWS), which is with a planar structure and is suitable for micro fabrication, is proposed for W-band traveling-wave tubes (TWT). Supported by a pair of T-shaped dielectric rods, the RRB SWS is fit for dual-sheet beam operation. The high frequency characteristics are analyzed by using computer simulations. Wide bandwidth input-output structures adopting tapered structure and step waveguide are designed. Hot-test performance of the RRB SWS is investigated by means of Particle-in-cell (PIC) simulations. A solenoid magnetic field of 0.6 T is adopted to focus the sheet beams with voltage and current of 11.2 kV and 0.12 A. The saturated output power of 56.7 W at 94 GHz is obtained at the output port, corresponding a gain of 27.4 dB. In addition, an attenuator is added to suppress oscillations and achieve stable operation.Introduction
As the development of radar detection technology,the requirement on target resolution ratio becomes higher and higher. Thus,millimeter wave radar system has attracted researcher’s attentions. W-band(75~110 GHz)is characterized by a large bandwidth and high resolution,which can be effectively utilized with lower atmospheric absorption,and it has an important application prospect in radar and satellite space communication [1]. The high-power devices in W-band are mainly vacuumed electron devices,including TWT [2],backward-wave oscillator(BWO)[3],gyrotron [4],etc.,among them TWT has been widely investigated for its wide bandwidth.
A slow wave structure is the core parts of a TWT. At W-band,the folded waveguide [5] has been the most popular slow wave structure for the advantages as high power and excellent heat dissipation. The cylindrical electron beam has been commonly used in folded waveguides,but as the structure size decreases,the cylindrical electron beam will suffer a strong space charge effect when the current is relatively large. Therefore,sheet electron beam becomes more and more popular with its weak space charge effect by increasing the width or height of the sheet beam.
There are several SWSs have been proposed and applied for W-band sheet-beam TWTs,including sine waveguide SWS [6],double-staggered grating SWS [7-8] and meander-line SWS [9-11]. In addition,the RRB SWS developed from the conventional ring-bar SWS is also suitable for sheet-beam TWT.
For the RRB SWS,reference [12] found that its ratio of backward-wave interaction impedance and forward-wave interaction impedance of the RRB SWS is lower than that of the planar helix with straight-edge connections(PH-SEC)SWS,indicating that the RRB SWS could operate more stability. In the reference [13],the RRB SWS based on dielectric substrates was presented,and its dispersion characteristics and interaction impedance were analyzed by using quasi-TEM approximation approach. The results show that the dispersion of the RRB SWS became weaker and the bandwidth became wider after adding dielectric substrates,and it has the characteristic of high gain.
In this paper,the DBRRB SWS is proposed for a W-band dual-sheet beam TWT. The high frequency characteristics have been investigated by using simulation. A wide band input-output structure has been proposed for the DBRRB SWS,leading to a good transmission curve. The hot performance of the DBRRB SWS,cooperating with two sheet electron beams,are obtained by using particle in cell(PIC)simulation.
1 High-frequency characteristics of the DBRRB SWS
Fig. 1 shows the single-period structure model of the DBRRB SWS,which consists of two rectangular rings and metal rods. The SWS is supported by a pair of T-shaped dielectric rods at the narrow sides. As a result,there are space for two sheet electron beams,locating along the broad sides. Although the inner tunnel of the rectangular ring has a higher interaction impedance than the outer side,the size of the inner tunnel is much smaller,leading to a higher current density and higher capture rate for electrons.
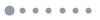
Figure 1.The structure of the DBRRB SWS with T-shaped dielectric rods supporting
For a TWT,the lower the normalized phase velocity of the SWS is,the lower the operation voltage will be. And the higher the interaction impedance is,the stronger the wave interaction will be. For the proposed SWS,H and W are the height and width of the rectangular ring,L is the length of the metal rod,ed is the vertical distance between the electron beam and the SWS. The single period length of the SWS is 2*(L+dt),when dt is determined,L has great influence on the high frequency characteristics of the SWS. The CST Eigen-mode solver and HFSS are used to analyze the high frequency characteristics of the SWS. Fig. 2 shows the effect of parameter L on interaction impedance and normalized phase velocity of the SWS by CST. When L decreases,the normalized phase velocity will decrease and the interaction impedance will increase,which means that reducing L can decrease the working voltage and increase the degree of beam-wave interaction. Besides,the phase velocity is not linearly proportional to the period length(vp1 /P1≠vp2 /P2,vp /P=2πf/φ)in the RRB SWS,for the reason of the phase shift changes with the period length,as shown in Fig. 3. The phase shift at 94 GHz increases by an average of 15° for every 0.04 mm increase in the period length.
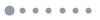
Figure 2.The interaction impedance(a)and normalized phase velocity,(b)vary with L
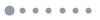
Figure 3.The f-phase curve with different L
W and H mainly affect the upper and lower cut-off frequencies of the fundamental mode of the slow-wave structure,and increasing W is beneficial to the focusing of the dual-sheet beam. When W increases,H needs to be reduced to keep the cut-off frequency of the fundamental mode consistent. Fig. 4 shows the variations of interaction impedance and normalized phase velocity with W/H,as can be seen,when W/H increases,the interaction impedance and normalized phase velocity will decrease.
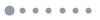
Figure 4.The interaction impedance(a)and normalized phase velocity,(b)vary with W/H
Fig. 5(a)shows the electric field distribution of the SWS along the direction of electron beam propagation in 94 GHz,which has axial field components,and the closer the electron beam to the SWS is,the stronger the electric field is and the higher the interaction impedance will be. From Fig. 5(b),ed varies from 0.04 mm to 0.06 mm,the interaction impedance is about 28 Ω to 18 Ω in 94 GHz. When ed is set to 0.04 mm,the interaction impedance is about 36~15 Ω from 89~100 GHz in CST. And the interaction impedance curves calculated by CST and HFSS have the same trend with a little difference.
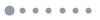
Figure 5.(a)Axial electric field distribution of the SWS in 94GHz,(b)interaction impedance varies with ed
When the structural parameters of the SWS are determined,the dispersion curve of the SWS is confirmed and shown in Fig. 6. The dispersion curves calculated by CST and HFSS are consistent,which verifies the rationality of the design of the structure. The operation voltage at 94 GHz is calculated as 10.6 kV. The 10.6 kV beam line intersects with mode 1 and mode 2 at 94 GHz of working frequency point and 130 GHz of backward-wave frequency point,respectively. The interaction impedance of the backward-wave frequency point is about 10 Ω,which may cause oscillation in the TWT.
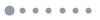
Figure 6.The dispersion curves of the SWS
2 Analysis of transmission characteristics
The simulation model of the whole TWT is shown in Fig. 7(a),which includes the input and output coupling structure,the slow wave line(Molybdenum plated with Copper,conductivity of 2.8×107 S/m),dielectric rods(BN,relative dielectric constant of 3.8,loss tangent of 0.005),the attenuator and two cathodes. The attenuator,as shown in Fig. 7(b),is designed to suppress backward-wave oscillation and reflection oscillation,the material is BeO with the relative dielectric constant of 6.5 and loss tangent of 0.5. The input-output coupling structure consists of two sections,one is the gradient structure,as shown in Fig. 7(c),which includes three periods of gradient structure with a height ratio of S. The other section is a step waveguide,as shown in Fig. 7(d),which transits the slow wave line to the WR-10 standard rectangular waveguide,and the size of WR-10 is 2.540 0 mm×1.270 0 mm.
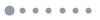
Figure 7.(a)The whole TWT structure,(b)attenuator,(c)three periods gradient structure,(d)three-segment step waveguide
The parameters of the TWT are listed in Table 1. The S-parameters of the step waveguide are investigated. The main parameters affecting the S-parameters of the step waveguide are gx1,gx2 and gx3,which can be optimized by adjusting the size and proportion between those parameters. From Fig. 8,we can see that the S11 of the step waveguide is lower than -15 dB in the whole W-band and the S21 is close to 0.
Parameter | Value | Parameter | Value |
---|
L | 0.04 | H | 0.46 |
W | 0.17 | dt | 0.03 |
dw | 0.03 | Dx | 0.80 |
Dy | 0.15 | gx1 | 0.81 |
gx2 | 0.38 | gx3 | 0.08 |
gy | 0.52 | L1 | 0.07 |
L2 | 0.50 | L3 | 1.00 |
Table 1. Parameters of the TWT (mm)
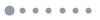
Figure 8.S-parameters of the step waveguide
The attenuator divides the whole slow wave line into three segments with period numbers of p1=60,p2=10 and p3=80,respectively. The S-parameters of the whole simulation model are simulated by CST time domain solver,and the results are shown in Fig. 9. The parameter S is the height gradient ratio of the gradient structure and S=1 means there is no gradient structure. When S=0.8,the S11 parameter becomes better than S=1,which means the gradient structure can effectively improve the S11 of the TWT. As can be seen,the S11 is lower than -20 dB in the range of 83~113 GHz. From Fig. 8(b),the S11 curves are almost the same with or without attenuator. The S21 without attenuator is -30 dB in 94 GHz duo to dielectric and metal loss,and after adding the attenuator the S21 is reduced to -60 dB.
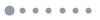
Figure 9.(a)S11 with different S of the TWT,(b)S-parameters with or without attenuator
3 Simulation of beam wave interaction
The beam wave interaction of the TWT is simulated by using CST particle-in-cell studio(CST-PIC). The PIC simulation of the TWT is carried out with the model in section-2,the attenuator has been added to suppress the possible oscillations,and good S-parameters have been obtained. Parameters that need to be optimized include the voltage and current of the electron beam,p3,ed,and the input power.
The height and width of the two identical cathodes are set as eh=0.4 mm and ew=0.08 mm respectively. And the vertical distance between the electron beam and the SWS is optimized to ed=0.04 mm,which is decided under the condition of electron interception loss and interaction impedance. The smaller the value of ed is,the stronger the interaction between the electron beam and the SWS is,and the higher the output power can be obtained. However,when it is too small,electrons will be captured by the SWS,resulting in electron interception loss. The optimized voltage and current of each electron beam are found as 11.2 kV and 0.06 A,respectively. The corresponding current density of each cathode is 187.5 A/cm2,which can be further decreased by increasing the width or height of the cathode.
In addition,the focusing magnetic field is set to 0.6 T,and the number of periods of the SWS is 150. The output signals of the SWS after beam wave interaction is shown in Fig. 10(a)as well as the input signal,from which the output power can be calculated as 56.7 W at 94 GHz with the input power of 0.1 W,corresponding a gain of 27.4 dB and electron efficiency of 4.2%. Besides,the output signals when there is no input signal have been investigated,as shown in Fig. 10(b). It is clear that there are no oscillations for at least 20 ns simulation,indicating that no oscillation occurs without input power.
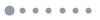
Figure 10.The time-domain output signals with(a)and without,(b)input power
Fig. 11 shows the spectrum of the ports’ signals,there is no indication of backward wave oscillation,as the reflected signal is significantly lower than the incident signal and the frequency spectrum of output signal is relatively clean. That is to say,the attenuator has effectively suppressed the oscillations. Fig. 12 shows electron beam bunching and phase space diagram,as the interaction length increases,the number of electrons in the deceleration zone gradually exceeds that in the acceleration zone,and the energy of electrons is gradually exchanged to the high frequency field. Besides,the TWT is operating at a linear region and close to saturation in length.
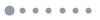
Figure 11.The spectrum of signals
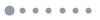
Figure 12.Electron beam bunching and phase space diagram
Fig. 13 shows the output power varies with beam width ew and beam height eh,while the beam current is retained. It can be seen that the increase of eh has little effect on output power. And the increase of ew has a greater impact on the output power,which is because of the interaction impedance decreases when goes far from the center of the slow wave structure. Fig. 14 shows the input-output power and gain curves,the saturated output power is 56.7 W when the input power of 0.1 W,and the gain decreases as the input power increases. The f-power and f-gain curves are shown in Fig. 15,the output power exceeds 20 W in the range of 91~96 GHz and the 3- dB bandwidth is close to 5 GHz.
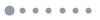
Figure 13.The output power varies with ew and eh
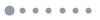
Figure 14.The input-output power and gain curves
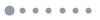
Figure 15.The output power and gain vary frequency
4 Conclusion
In this paper,the DBRRB SWS with T-shape dielectric rods supporting has been proposed for a W-band TWT. Good transmissions have been obtained with the addition of the gradient structure and the step waveguide. The oscillations were well suppressed by the addition of an attenuator. The beam wave interaction results show that the saturated output power is 56.7 W and the gain is 27.4 dB at 94 GHz under the condition of two sheet beams(11.2 kV,0.12 A),0.6 T focusing magnetic field and the input power of 0.1 W. The output power exceeds 20 W in the range of 91~96 GHz.
References
[1] A Jebril, M Lucente, T Rossi et al. New developments in the WAVE W-band mission(2006).
[2] A J Theiss, C J Meadows, R Freeman et al. High-Average-Power W-band TWT Development. IEEE TRANSACTIONS ON PLASMA SCIENCE, 38, 1239-1243(2010).
[3] M M A Kumar, S Aditya, Shao-Meng WANG. A W-band backward-wave oscillator based on planar helix slow wave structure. IEEE TRANSACTIONS ON ELECTRON DEVICES, 65, 5097-5102(2018).
[4] H H Song, D B Mcdermott, Y Hirata et al. Theory and experiment of a 94 GHz gyrotron traveling-wave amplifier. PHYSICS OF PLASMAS, 11, 2935-2941(2004).
[5] Jin-Jun FENG, Jun CAI, Yin-Fu HU et al. Development of w-band folded waveguide pulsed TWTs. IEEE TRANSACTIONS ON ELECTRON DEVICES, 61, 1721-1725(2014).
[6] S Z FANG, J XU, X LEI et al. Design of a W-band traveling-wave tube based on sine waveguide slow-wave structure with sheet electron beam(2019).
[7] Zhi-Gang LU, Rui-Dong WEN, Wei-Hua GE et al. Design of a low-gain high-power w-band sheet-beam traveling wave tube using a double-staggered grating slow wave structure. JOURNAL OF ELECTROMAGNETIC WAVES AND APPLICATION, 33, 1996-2008(2019).
[8] Jian-Qiang LAI, Yu-Bin GONG, Xiong XU et al. W-band 1-kw staggered double-vane traveling-wave tube. IEEE TRANSACTIONS ON ELECTRON DEVICES, 59, 496-503(2012).
[9] S Sengele, Hong-rui Jiang, J H Booske et al. Microfabrication and characterization of a selectively metallized w-band meander-line twt circuit. IEEE TRANSACTIONS ON ELECTRON DEVICES, 56, 730-737(2009).
[10] Fei SHEN, Yan-Yu WEI, Hai-Rong YIN et al. A novel v-shaped microstrip meander-line slow-wave structure for w-band mmpm. IEEE TRANSACTIONS ON PLASMA SCIENCE, 40, 463-469(2012).
[11] Fei SHEN, Yan-Yu WEI, Xiong XU et al. Symmetric double v-shaped microstrip meander-line slow-wave structure for w-band traveling-wave tube. IEEE TRANSACTIONS ON ELECTRON DEVICES, 59, 1551-1557(2012).
[12] C Chua, S Aditya, J M Tsai et al. Microfabricated Planar Helical Slow-Wave Structures Based on Straight-Edge Connections for THz Vacuum Electron Devices. Terahertz Science and Technology, 4, 208-229(2011).
[13] Cheng-fang FU, Bo ZHAO, Yu-dong YANG et al. Slow-Wave Characteristics of a Frame–Rod Structure Based on Micro-Fabricated Technology for THz Vacuum Electron Devices. Journal of Infrared Millimeter & Terahertz Waves, 37, 1106-1116(2016).