Author Affiliations
1School of Chemical Science and Technology, Yunnan University, Kunming 650500, China2Center for Excellence in Nanoscience (CAS), Key Laboratory of Nanosystem and Hierarchical Fabrication (CAS), National Center for Nanoscience and Technology, Beijing 100190, Chinashow less
Organic electrochemical transistors (OECTs) have attracted attention due to their unique function of converting ionic and biological signals into electronic signals, high transconductance, low energy consumption (below 1 V), stable operation in aqueous media, good biocompatibility[1, 2]. However, most OECTs are usually built on brittle and stiff substrates, and inappropriate to be adhered to or contacted with delicate human skin, thus impeding their use in wearable electronics. It is desirable to exploit stretchable OECTs to reduce the mechanical mismatch with soft tissues.
Stretchable OECTs can be divided into intrinsically stretchable OECTs and structurally stretchable OECTs (Fig. 1), depending on the strategy adopted for establishing its stretchability. Intrinsically stretchable OECTs require that all OECT’s components are inherently stretchable[3]. Since the performance of OECTs has an inherent dimension dependence, the deformation affects output signals significantly[4]. In contrast, structurally stretchable OECTs have a low requirement for intrinsic stretchability for each device components, and the stretchability can be obtained through device structure engineering. Structurally stretchable OECTs exhibit good stretchability and high performance along with steady output signal under deformation, demonstrating potential applications in wearable electronics. Micro- and nano-structures have been introduced into the substrate, electrode, semiconductor and device for developing structurally stretchable OECTs (Fig. 2).
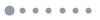
Figure 1.(Color online) Stretchable OECTs.
![(Color online) (a) Micro-/nano-structured substrate. Reproduced with permission[5, 6], Copyright 2019 and 2021, Wiley-VCH. (b) Micro-/nano-structured electrode. Reproduced with permission[8], Copyright 2018, Science (AAAS). Reproduced with permission[9], Copyright 2019, Wiley-VCH. (c) Micro-/nano-structured organic semiconductors. Reproduced with permission[10], Copyright 2012, American Chemical Society. Reproduced with permission[12], Copyright 2018, Science (AAAS). (d) Micro-/nano-structured device. Reproduced with permission[16], Copyright 2017, American Chemical Society. Reproduced with permission[13], Copyright 2022, Nature Publishing Group.](/Images/icon/loading.gif)
Figure 2.(Color online) (a) Micro-/nano-structured substrate. Reproduced with permission[5, 6], Copyright 2019 and 2021, Wiley-VCH. (b) Micro-/nano-structured electrode. Reproduced with permission[8], Copyright 2018, Science (AAAS). Reproduced with permission[9], Copyright 2019, Wiley-VCH. (c) Micro-/nano-structured organic semiconductors. Reproduced with permission[10], Copyright 2012, American Chemical Society. Reproduced with permission[12], Copyright 2018, Science (AAAS). (d) Micro-/nano-structured device. Reproduced with permission[16], Copyright 2017, American Chemical Society. Reproduced with permission[13], Copyright 2022, Nature Publishing Group.
A biomimicking polydimethylsiloxane (PDMS) film with 2D wavy microstructure, transferred from the bract of Bougainvillea glabra, was reported by Yan et al. in 2019[5]. By virtue of the 2D wavy microstructure surface, the actual strain applied to the device is substantially alleviated, resulting in a device-level omnidirectional stretchability. The corresponding OECTs showed a stable performance at a strain of 30% and a bending radius of 15 mm, and were successfully used in an attachable glucose sensor with a detection limit of 1 μM. On a 3D mogul-patterned PDMS substrate, Lee et al. fabricated stretchable and stable electrolyte-gated OECTs[6]. In this device, bumps and valleys were regularly positioned to form a hexagonal close-packed structure. Such a 3D mogul microstructure could efficiently absorb stretching tension in the lateral direction with minimal stress concentration, thus gifting good stretchability. The resultant OECTs showed high durability under 30% stretching.
Various stretchable conductors have been developed such as Ag nanowire, carbon nanotubes, and liquid metals. Stretchable Au electrode is also desirable for OECTs due to its good stability and compatibility with the electrolyte. However, dense Au thin films are not stretchable, while those with certain micro-/nano-structure might be. Because the particular line can function like a spring, and unfold progressively along with out-of-plane deformation upon applying strain, the horseshoe shape or serpentine structure is commonly used to enhance the stretchability of non-stretchable materials. Ramuz et al. made a stretchable OECT with horseshoe-shape Au/Al electrode, which was patterned by laser cutting and encapsulated in PDMS[7]. The device showed a good performance under 11% strain, revealing a maximum stretchability of 38% and a transconductance of 0.35 mS. Someya et al. fabricated an ultrathin, stretchable OECT on a honeycomb grid Au substrate. An excellent electrical property and mechanical stretchability was achieved with only 7% loss in transconductance and source-drain current values under 15% strain[8]. The stretchability was enabled by the intrinsic deformation of honeycomb structures. A stretchable and blood-compatible OECT array was further integrated for in vivo recording of electrocardiogram (ECG) signals of a rat heart. An irregular microcrack morphology was also proved to increase the stretchability of Au conductors by Chen et al.[9]. They discovered that initial short microcracks (<2 μm) may easily propagate into longer microcracks and disrupt the conducting pathways, while initial longer microcracks (>8 μm) can accommodate larger strains and suppress microcrack propagation. By using highly stretchable Au conductors with controlled microcracks, the device exhibited a high transconductance under 0% strain (0.54 mS) and 140% strain (0.14 mS), and was further utilized in stretchable synaptic devices. The stretchable OECTs as sensors can be implanted onto living organs for continuous monitoring.
Controlling the 2D/3D micro-/nano-structures is an alternative strategy for improving the stretchability, such as nano-arch, nano-fiber and porous morphology. Margaritondo et al. developed an accurate 3D writing technique to make highly stretchable organic nanowire arrays with a 3D nano-archy structure, showing a stretchability of over 270% while maintaining electrical property[10]. It is challenging for biointerface applications due to the non-coplanar device configuration. The chain entanglement can boost the stretchability even for stiff semiconducting polymers. Cicoira et al. made highly stretchable conjugated polymer nanofibers via an electrospinning coating method[11]. The fibers could be stretched to 140% while losing just 16% of their conductivity. In addition, stretchable OECTs with polymer fibers as the active layer showed transistor behavior up to ~50% strain. Also, Lee et al. reported a stretchable OECT for optoelectronic sensorimotor synapse with organic nanowire as the channel material[12]. The maximum drain current and carrier mobility was unaffected under 100% strain for multiple cycles. Moreover, Facchetti et al. demonstrated that the stretchability of honeycomb-structured film was greater than that of dense film, as proven by both simulation and laboratory findings[13]. In this context, a variety of stretchable OECTs have been reported based on multilayer porous polymer films[14, 15].
Wavy structure is often achieved by pre-stretching, and all the device components are wrinkled and compressed after releasing the pre-stretched tension. The wavy structure allows the device to be stretched without significant degradation of the electrical performance. Cicoira et al. made stretchable OECTs by first pre-stretching PDMS substrate, then patterning Au source/drain and channel materials through transfer and orthogonal patterning, and finally relaxing the pre-strain[16]. The devices displayed a high stretchability with identical transconductance under 0%−30% strain. Facchetti et al. developed a stretchable OECT based on honeycomb polymer semiconductor and biaxially-pre-stretched platform[13]. An excellent stretchability and stable electrical performance were presented during a biaxial stretching of 30%−140%. A mechanical robustness was demonstrated with a small degradation of the maximum transconductance under 30% strain.
In short, we highlight the structurally stretchable OECTs with micro-/nano-structures in the substrates, electrodes, semiconductors, and devices. They can deliver a stable signal during deformation, and they will find applications in wearable electronics.
References
[1] W Huang, J H Chen, Y Yao et al. Vertical organic electrochemical transistors for complementary circuits. Nature, 613, 496(2023).
[2] J Rivnay, S Inal, A Salleo et al. Organic electrochemical transistors. Nat Rev Mater, 3, 17086(2018).
[3] Y Yao, W Huang, J H Chen et al. Flexible and stretchable organic electrochemical transistors for physiological sensing devices. Adv Mater, 35, 2209906(2023).
[4] V G Reynolds, S Oh, R X Xie et al. Model for the electro-mechanical behavior of elastic organic transistors. J Mater Chem C, 8, 9276(2020).
[5] Y Z Li, N X Wang, A N Yang et al. Biomimicking stretchable organic electrochemical transistor. Adv Electron Mater, 5, 1900566(2019).
[6] T D Nguyen, T Q Trung, Y Lee et al. Stretchable and stable electrolyte-gated organic electrochemical transistor synapse with a nafion membrane for enhanced synaptic properties. Adv Eng Mater, 24, 2100918(2021).
[7] B Marchiori, R Delattre, S Hannah et al. Laser-patterned metallic interconnections for all stretchable organic electrochemical transistors. Sci Rep, 8, 8477(2018).
[8] W Lee, S Kobayashi, M Nagase et al. Nonthrombogenic, stretchable, active multielectrode array for electroanatomical mapping. Sci Adv, 4, eaau2426(2018).
[9] N Matsuhisa, Y Jiang, Z Y Liu et al. High-transconductance stretchable transistors achieved by controlled gold microcrack morphology. Adv Electron Mater, 5, 1900347(2019).
[10] J T Kim, J Pyo, J Rho et al. Three-dimensional writing of highly stretchable organic nanowires. ACS Macro Lett, 1, 375(2012).
[11] M Lerond, A Subramanian, W G Skene et al. Combining electrospinning and electrode printing for the fabrication of stretchable organic electrochemical transistors. Front Phys, 9, 708914(2021).
[12] Y Lee, J Y Oh, W Xu et al. Stretchable organic optoelectronic sensorimotor synapse. Sci Adv, 4, eaat7387(2018).
[13] J H Chen, W Huang, D Zheng et al. Highly stretchable organic electrochemical transistors with strain-resistant performance. Nat Mater, 21, 564(2022).
[14] C J Liu, J Y Deng, L Gao et al. Multilayer porous polymer films for high-performance stretchable organic electrochemical transistors. Adv Electron Mater, 9, 2300119(2023).
[15] Y J Peng, L Gao, C J Liu et al. Stretchable organic electrochemical transistors via three-dimensional porous elastic semiconducting films for artificial synaptic applications. Nano Res, 16, 10206(2023).
[16] S M Zhang, E Hubis, G Tomasello et al. Patterning of stretchable organic electrochemical transistors. Chem Mater, 29, 3126(2017).