
- Chinese Optics Letters
- Vol. 19, Issue 10, 102602 (2021)
Abstract
1. Introduction
The photonic nanojet (PNJ), which was first discovered by Chen et al. in 2004[
The formation of a PNJ is a complex process that involves refraction, interference, and diffraction of an electromagnetic wave incident on a dielectric particle at the wavelength scale. By tuning the diameter and refractive index of the illuminated dielectric structure (customarily assumed to be a cylinder or sphere), the properties of the generated PNJ, including the maximum intensity, PNJ length, and full width at half-maximum (FWHM), can be greatly modified[
However, while various dielectric structures have been investigated, the incident light source of PNJs in most studies has been restricted to a simple plane wave. If we attribute the generation of a PNJ to a process analogous to the jet-like nano-focusing in the near-field region[
Sign up for Chinese Optics Letters TOC. Get the latest issue of Chinese Optics Letters delivered right to you!Sign up now
In this Letter, we propose and numerically investigate a novel PNJ generated by a circularly polarized vortex beam incident on a dielectric microsphere. The PNJ appears as a donut-like focal field with longitudinal reverse energy flow; such a flow could greatly enhance capabilities in the fields of optical micromanipulation and trapping. The dependence of the reverse energy flow of the donut-like PNJ on both the topological charge of the vortex beam and the geometry of the microsphere is investigated in detail. The unique properties of the PNJ are similar to those associated with vector diffraction of an incident light beam focused by a high numerical aperture (NA) lens, a problem that can be treated analytically using the Richards–Wolf (RW) integral.
2. Results and Discussions
Figure 1 shows schematically a donut-like PNJ generated by a dielectric microsphere with radius
Figure 1.Schematic of the generation of a donut-like photonic nanojet (PNJ). Incident light has circular polarization σ and topological charge m.
The electric field intensity distribution
Figure 2.Electric field intensity distribution |E|2 of the PNJ generated by a dielectric sphere (R = λ) under illumination by left circularly polarized Laguerre–Gaussian beams with topological charges (a) m = 2 and (b) m = 0. For each case, the top plot is the |E|2 of the PNJ, while the bottom one is the longitudinal intensity profile. The inset is the transverse profile with marked full width at half-maximum, corresponding to the dashed red line that crosses the maximum intensity point of the PNJ. (c) and (d) plot |E|2 at the focal planes of the PNJs in (a) and (b), respectively.
Another unique property of the proposed donut-like PNJ is the appearance of reverse energy flow along the optical axis in the vicinity of the focal plane. We investigate the PNJ generated by microspheres with radii ranging from
Figure 3.Longitudinal energy flow Pz at the focal plane of the PNJs generated by left circularly polarized Laguerre–Gaussian beams with (a) fixed topological charge m = 2 and refractive index n = 1.5 but different microsphere radii R; (b) fixed m = 2, R = 6λ but different n; and (c) fixed n = 1.5, R = 6λ but different m. Insets: Pz at x = 6.
To understand better the reverse
Figure 4.Energy flow of the PNJs generated by a dielectric sphere (R = 6λ, n = 1.5) illuminated by left circularly polarized Laguerre–Gaussian beams with topological charges (a) m = 2 and (b) m = 0, corresponding to Figs.
So far, our investigation of the reverse energy flow in the donut-like PNJ has been numerical, but a more analytical approach is also possible and preferable. PNJ generation can be viewed as analogous to the tight-focusing process of a high NA lens, which can also exhibit reverse energy flow when the incident light is a circularly polarized vortex beam[
The longitudinal component of the Poynting vector is then
For the LCP-LG light beam with
Figure 5.Longitudinal component of the Poynting vector along the x axis in the focal plane of the proposed donut-like PNJ (red curve) compared to that predicted from the RW integral (blue curve). The incident light is a left circularly polarized Laguerre–Gaussian beam with topological charge m = 2. The dielectric microsphere has R = 6λ and n = 1.5, and the NA of the lens is one.
3. Conclusion
In conclusion, we propose and investigate a novel donut-like PNJ formed by illuminating a dielectric microsphere with a circularly polarized vortex beam. We numerically study the dependence of PNJ properties on the vortex topological charge and the geometry and refractive index of the microsphere, demonstrating the subwavelength hollow-ring shape of the PNJ field and the existence of longitudinal reverse energy flow at the focal plane. In addition, we explain this unique PNJ property by an approximation of the tight-focusing process, analytically modeled with the RW vector-diffraction integral. The donut-like PNJ, with its structured focal field and unusual energy-flow distribution, may be applicable to novel optical trapping and micromanipulation.
References
[1] Z. Chen, A. Taflove, V. Backman. Photonic nanojet enhancement of backscattering of light by nanoparticles: a potential novel visible-light ultramicroscopy technique. Opt. Express, 12, 1214(2004).
[2] Z. B. Wang, W. Guo, L. Li, B. Luk’yanchuk, A. Khan, Z. Liu, Z. C. Chen, M. H. Hong. Optical virtual imaging at 50 nm lateral resolution with a white-light nanoscope. Nat. Commun., 2, 218(2011).
[3] A. Darafsheh, C. Guardiola, A. Palovcak, J. C. Finlay, A. Carabe. Optical super-resolution imaging by high-index microspheres embedded in elastomers. Opt. Lett., 40, 5(2015).
[4] G. Huszka, H. Yang, M. A. M. Gijs. Microsphere-based super-resolution scanning optical microscope. Opt. Express, 25, 15079(2017).
[5] D. Gerard, J. Wenger, A. Devilez, D. Gachet, B. Stout, N. Bonod, E. Popov, H. Rigneault. Strong electromagnetic confinement near dielectric microspheres to enhance single-molecule fluorescence. Opt. Express, 16, 15297(2008).
[6] P. K. Upputuri, M. Pramanik. Microsphere-aided optical microscopy and its applications for super-resolution imaging. Opt. Commun., 404, 32(2017).
[7] F. Wang, S. Yang, H. Ma, P. Shen, N. Wei, M. Wang, Y. Xia, Y. Deng, Y.-H. Ye. Microsphere-assisted super-resolution imaging with enlarged numerical aperture by semi-immersion. Appl. Phys. Lett., 112, 023101(2018).
[8] E. McLeod, C. B. Arnold. Subwavelength direct-write nanopatterning using optically trapped microspheres. Nat. Nanotech., 3, 413(2008).
[9] A. Jacassi, F. Tantussi, M. Dipalo, C. Biagini, N. Maccaferri, A. Bozzola, F. De Angelis. Scanning probe photonic nanojet lithography. ACS Appl. Mater. Interfaces, 9, 32386(2017).
[10] Y. C. Li, H. B. Xin, H. X. Lei, L. L. Liu, Y. Z. Li, Y. Zhang, B. J. Li. Manipulation and detection of single nanoparticles and biomolecules by a photonic nanojet. Light: Sci. Appl., 5, e16176(2016).
[11] A. Shakhov, A. Astafiev, V. Nadtochenko. Microparticle manipulation using femtosecond photonic nanojet-assisted laser cavitation. Opt. Lett., 43, 1858(2018).
[12] Y. C. Li, H. B. Xin, X. S. Liu, Y. Zhang, H. X. Lei, B. J. Li. Trapping and detection of nanoparticles and cells using a parallel photonic nanojet array. ACS Nano, 10, 5800(2016).
[13] S. C. Kong, A. V. Sahakian, A. Taflove, V. Backman. Photonic nanojet-enabled optical data storage. Opt. Express, 16, 13713(2008).
[14] S. C. Kong, A. V. Sahakian, A. Heifetz, A. Taflove, V. Backman. Robust detection of deeply subwavelength pits in simulated optical data-storage disks using photonic jets. Appl. Phys. Lett., 92, 211102(2008).
[15] A. Heifetz, S. Kong, A. V. Sahakian, A. Taflove, V. Backman. Photonic nanojets. J. Comput. Theoretical Nanos., 6, 1979(2009).
[16] B. S. Luk’yanchuk, R. Paniagua-Domínguez, I. Minin, O. Minin, Z. Wang. Refractive index less than two: photonic nanojets yesterday, today and tomorrow. Opt. Mater. Express, 7, 1820(2017).
[17] Y. C. Shen, L. H. V. Wang, J. T. Shen. Ultralong photonic nanojet formed by a two-layer dielectric microsphere. Opt. Lett., 39, 4120(2014).
[18] G. Q. Gu, R. Zhou, Z. C. Chen, H. Y. Xu, G. X. Gai, Z. P. Gai, M. H. Hong. Super-long photonic nanojet generated from liquid-filled hollow microcylinder. Opt. Lett., 40, 625(2015).
[19] B. F. Zhang, J. J. Hao, Z. Shen, H. Wu, K. Zhu, J. Xu, J. P. Ding. Ultralong photonic nanojet formed by dielectric microtoroid structure. Appl. Opt., 57, 8331(2018).
[20] L. Y. Yue, O. V. Minin, Z. B. Wang, J. N. Monks, A. S. Shalin, I. V. Minin. Photonic hook: a new curved light beam. Opt. Lett., 43, 771(2018).
[21] S. Zhou. Twin phonotic hooks generated from two coherent illuminations of a micro-cylinder. J. Opt., 22, 085602(2020).
[22] I. V. Minin, O. V. Minin, G. M. Katyba, N. V. Chernomyrdin, V. N. Kurlov, K. I. Zaytsev, L. Yue, Z. Wang, D. N. Christodoulides. Experimental observation of a photonic hook. Appl. Phys. Lett., 114, 031105(2019).
[23] H. Guo, Y. Han, X. Weng, Y. Zhao, G. Sui, Y. Wang, S. Zhuang. Near-field focusing of the dielectric microsphere with wavelength scale radius. Opt. Express, 21, 2434(2013).
[24] T. Zeng, J. Ding. Three-dimensional multiple optical cages formed by focusing double-ring shaped radially and azimuthally polarized beams. Chin. Opt. Lett., 16, 031405(2018).
[25] Z. Gu, X. Wang, J. Wang, F. Fan, S. Chang. Sidelobe suppression and axial resolution enhancement in 4pi microscopy with higher-order radially polarized Laguerre–Gaussian beams using subtractive imaging. Chin. Opt. Lett., 17, 121103(2019).
[26] J. Wang, X. Wang, M. Zeng. Broadband transverse displacement sensing of silicon hollow nanodisk under focused radial polarization illumination in the near-infrared region. Chin. Opt. Lett., 18, 063602(2020).
[27] V. V. Kotlyar, A. A. Kovalev, A. G. Nalamov. Energy density and energy flux in the focus of an optical vortex: reverse flux of light energy. Opt. Lett., 43, 2921(2018).
[28] V. V. Kotlyar, A. G. Nalimov, A. A. Kotlyar. Helical reverse flux of light of a focused optical vortex. J. Opt., 20, 095603(2018).
[29] B. Richards, E. Wolf. Electromagnetic diffraction in optical systems, II. Structure of the image field in an aplanatic system. Proc. R. Soc. A, 253, 358(1959).
[30] M. Born, E. Wolf. Principles of Optics(1999).
[31] M. V. Berry. Optical currents. J. Opt. A, 11, 094001(2009).
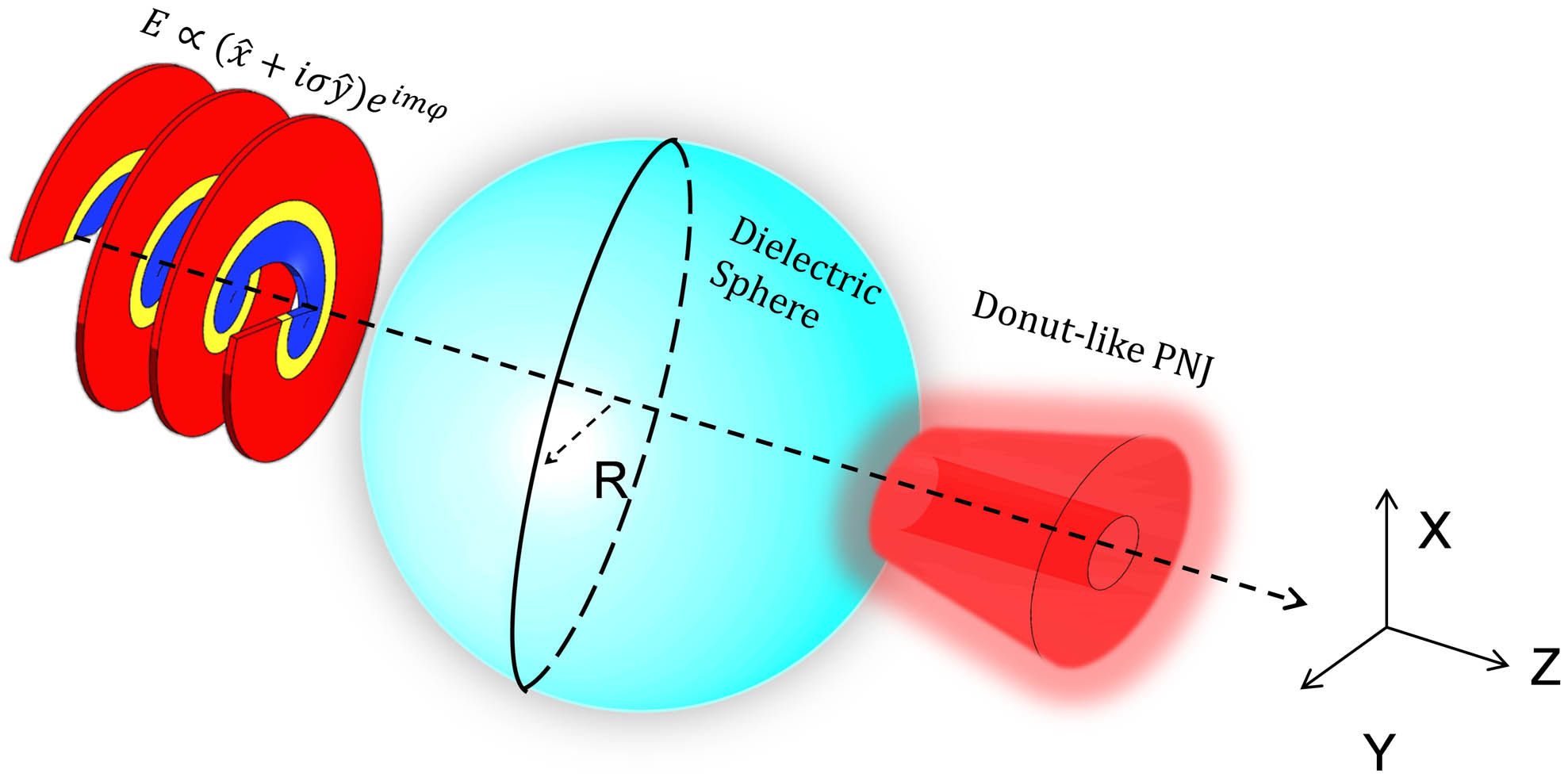
Set citation alerts for the article
Please enter your email address