Abstract
Optical frequency combs, which are generated by the cascade of a phase modulator and a Mach–Zehnder intensity modulator, are used as a polychromatic signal source in the terahertz imaging system to improve imaging quality. The interference effect caused by the monochromatic wave has been greatly suppressed. The required optical power in the presented system is as low as of that in the system using the Er-doped fiber amplifier as a source, which can reduce cost and protect photodiodes from damage. This work provides an effective, low power consumption, low cost, and easy way to realize terahertz imaging with high quality and can be used in future security inspections.Imaging with electromagnetic radiation at the terahertz (THz) frequency band (0.1–10 THz) is a promising technology for applications in biomedical imaging, material characterizations, and security screening[1–3]. Photonics-based technology has been attractive and developed to generate and distribute the THz signal in recent years[4]. In THz imaging systems based on photonics technology, due to low transmission loss of fiber[5], the generator could be located far from the antenna, and signal generation and processing schemes do not need to be centralized, decreasing the difficulty of configuration and development of the system.
However, when a monochromatic wave is used as the source for imaging systems, the interference effect, which is caused by a reflection of waves among mirrors and surfaces of objects under inspection, usually deteriorates imaging quality and spatial resolution. In Ref. [6], an Er-doped fiber amplifier (EDFA) was adopted as an amplified spontaneous emission (ASE) noise source to reduce the interference effect. Due to the ultra-broad bandwidth of ASE noise () and limited bandwidth of the photodiode, much ASE noise energy is wasted and cannot be effectively converted into a THz signal. Furthermore, when higher THz power is required to image objects with low transmittance, such extra ASE noise power can heat the photodiode, degrade device performance, and even burn out the photodiode.
In this Letter, we experimentally demonstrate a THz imaging system based on photonics technology operating at the band, where optical frequency combs are employed instead of EDFA as the signal source. Since multiple comb lines function as a polychromatic source, the interference effect can be largely suppressed compared with that obtained by a monochromatic wave signal source, and an image with high quality of wrench sandwiched between two thick cardboards was obtained. Moreover, the required optical input power in the presented system is as low as of that in the imaging system using an EDFA noise source.
Sign up for Chinese Optics Letters TOC. Get the latest issue of Chinese Optics Letters delivered right to you!Sign up now
In a monochromatic wave imaging system, the THz signal is generated by optical heterodyning of two wavelengths of light from two wavelength-tunable lasers, while an optical frequency comb generation part is added in the proposed imaging system, as shown in Fig. 1. Two 100-GHz-spaced wavelengths of light were first combined in an optical coupler and modulated through a cascaded phase modulator (PM) and a Mach–Zehnder modulator (MZM) driven by a sinusoidal radio frequency (RF) source. When we tune the DC bias voltages and the signal power of the RF source is applied on the cascaded PM and MZM (), multiple comb lines or peaks can be generated in a fixed frequency spacing () with different amplitudes[7]. Figure 2 shows the measured optical spectrum after cascaded PM and MZM under different driving conditions. Another MZM was used to allow for lock-in detection with a frequency of 50 kHz. Subsequently, the optical frequency comb was boosted by an EDFA. In our experiment, a commercially available uni-traveling carrier photodiode (UTC-PD) operating at [8] was employed as the THz photomixing emitter, realizing the photonic to THz wave conversion.
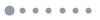
Figure 1.Experimental configuration of the THz imaging system. LD, laser diode; PM, phase modulator; MZM, Mach–Zehnderintensity modulator; RF, radio frequency source; UTC-PD, uni-traveling photodiode; LA, locked-in amplifier; SBD, Schottky barrier diode; PC, personal computer; PM1, PM2, PM3, and PM4, parabolic mirrors.
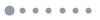
Figure 2.Output spectra of the experimentally generated combs when (a) and DC bias voltage of is applied on the MZM. (b) and DC bias voltage of is applied on the MZM.
The emitted THz radiation was collected by a pair of 90° off-axis parabolic mirrors (PM1 and PM2) and then focused onto the sample. The sample was placed at the focal plane of PM2 and PM3 and mounted on a computer-controlled X–Y translation stage. PM3 and PM4 guided the THz beams to the Schottky barrier diode (SBD, Millitech, DET-08, operating at ). The received signal was read out by a locked-in amplifier (LA) with the integration time 1 ms. A personal computer (PC) was used for collecting the transferred data from the LA and for controlling the translation stage. In order to simulate the scenario of security screening, such as express parcel inspection, a wrench sandwiched between two thick cardboards was selected as the sample for the imaging experiment, as shown in Fig. 3.
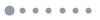
Figure 3.Pictures of the sample under inspection: (a) the wrench, (b) the wrench sandwiched between two thick cardboards (each thick).
Figure 4 depicts images obtained by the THz imaging system with different optical sources. The scanning time was about 2 min with an imaging area of . The scanning speed is mainly limited by the translation scan mechanism. For comparison, the image obtained by using the monochromatic wave source is shown in Fig. 4(a). Although the wrench can be identified, part of the edge of the wrench is blurred and not resolved, and many interference stripes appear in the background caused by the interference effect. The imaging quality will get worse when the thinner sample with higher transmittance is inspected.
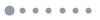
Figure 4.Images of the wrench obtained by different optical sources. (a) Monochromatic source where two wavelengths are separated by . (b) Optical frequency combs with and DC bias voltage of . (c) Optical frequency combs with and DC bias voltage of . (d) Optical frequency combs with and DC bias voltage of .
For an imaging system using optical frequency combs, multiple optical beat notes are generated by heterodyning among comb lines, which can be converted into THz waves at the band by the UTC-PD. Therefore, optical frequency combs can function as a polychromatic source, and the influence of the interference effect mainly caused by using a monochromatic wave source can be weakened greatly. By properly tuning the frequency and output power of the RF source and bias voltages applied on modulators, the images with high quality are obtained, as shown in Figs. 4(b) and 4(c) (frequency and 8 GHz, respectively). The edge of the wrench is distinguished, and interference stripes are significantly reduced. The small misalignment in Fig. 4(c) is caused by the delay of software recording during the movement of the translation stage. However, as becomes larger, most optical beat notes are out of the band; thus, fewer THz waves are generated, and the imaging quality gets worse, as demonstrated in Fig. 4(d), where .
Although the imaging system with an EDFA noise source can also effectively suppress the interference effect[6], as mentioned earlier, owing to the ultra-broad bandwidth of ASE noise and limited bandwidth of UTC-PD and SBD (), much ASE noise energy cannot be converted into a THz wave and just heat the UTC-PD, which is harmful for the UTC-PD and leads to high cost. For example, to obtain the same voltage at the LA (), the optical power launching into the UTC-PD is only 1.38 dBm when the optical frequency combs source is used, as low as of that for systems using the EDFA source (). The optical efficiency of the quantum cascade laser (QCL) is limited[9], about 1%, the power consumption of the modulator is very low, and the optical efficiency is much higher than that of the QCL. Imaging quality for the imaging system using optical frequency combs can be further improved by employing schemes generating more comb lines with high spectral flatness, like in Refs. [10,11], where more optical components are included.
In this work, a THz imaging system based on photonics technology operating at the band was demonstrated, where optical frequency combs were employed as the signal source. The influence of the interference effect was greatly reduced by using such a polychromatic source, compared with that obtained by a monochromatic wave source, and an image with high quality was obtained. The required optical power is as low as of that in the imaging system using an EDFA noise source. The presented system takes advantage of being easy, low power consumption, and low cost, and can be easily extended to other THz frequency bands to realize THz imaging of high quality for future security inspections or other applications.
References
[1] W. L. Chan, J. Deibel, D. M. Mittleman. Rep. Prog. Phys., 70, 1325(2007).
[2] H. Zhang, Z. Zhang, X. Zhao, X. Zhang, T. Zhang, C. Cao, Y. Yu. Chin. Opt. Lett., 16, 103001(2018).
[3] X. Xu, Y. Wu, T. He, Y. Li, F. Hu, H. Liang, H. Zhong. Chin. Opt. Lett., 15, 111703(2017).
[4] T. Nagatsuma, G. Ducournau, C. C. Renaud. Nat. Phonton., 10, 371(2016).
[5] A. Kanno, T. Kawanishi. J. Lightwave Technol., 32, 3566(2014).
[6] T. Nagatsuma, T. Kumashiro, Y. Fujimoto, K. Taniguchi, K. Ajito, N. Kukutsu, T. Furuta, A. Wakatsuki, Y. Kado. International Conference on Infrared Millimeter and Terahertz Waves, M3C05(2009).
[7] M. Fujiwara, M. Teshima, J. Kani, H. Suzuki, N. Takachio, K. Iwatsuki. J. Lighwave Technol., 21, 2705(2003).
[8]
[9] B. S. Williams, S. Kumar, Q. Hu, J. L. Reno. Electron. Lett., 42, 89(2006).
[10] R. Wu, R. Supradeepa, C. M. Long, D. E. Leaird, A. M. Weiner. Opt. Lett., 35, 3234(2010).
[11] J. Yu, Y. Li, F. Zhang, J. Wu, X. Hong, K. Xu, W. Li, J. Lin. Chin. Opt. Lett., 10, 042501(2012).