Abstract
A depolarization phenomenon in an electro-optical crystal in a quasi-three-level 946 nm Nd:YAG laser is observed. A compensation of the thermal effects in electro-optical crystals is achieved by employing a quarter-wave plate, with one optical axis parallel to the laser polarization. This technique allows for the production of an electro-optically -switched 946 nm Nd:YAG laser at 1 kHz. A maximum output power of 350 mW at 1 kHz repetition frequency and 11 ns pulse duration are achieved with an output coupler of 10% transmission under the incident pump power of 11.1 W, corresponding to a peak power of .Lasers from Nd:YAG with a quasi-three-level transition at 946 nm have been presented in Refs. [1–5]. Harmonic generation with nonlinear optical crystals of continuous-wave 946 nm lasers has numerous applications, such as in high-density optical data storage, flow cytometry, and color displays[6–8]. However, high-repetition-rate lasers with pulse widths of several nanoseconds are desirable for remote sensing and underwater communication. Such types of lasers can be obtained using acousto-optically[9–11] or electro-optically (E-O) -switching techniques. The latter can provide narrow pulses as well as linearly polarized laser beams, whereas the available data for E-O -switching is very limited. An E-O -switched 946 nm Nd:YAG laser was reported[12]. A maximum output energy of 24 mJ was achieved with a pulse width of 35 ns. However, the pulse repetition rate was only 2.5 Hz. The frequency was subsequently to 100 Hz[13]. A pulse energy of 3.2 mJ in an E-O -switched 946 nm Nd:YAG laser with a double-crystal (RTP) Pockel’s cell was obtained[13]. Up until now, the maximum pulse repetition rate of the E-O -switched 946 nm Nd:YAG lasers was no more than 100 Hz. In some applications, laser repetition of at least 1 kHz is needed. Nd:YAG lasers with a 1 kHz E-O-switched 946 nm Nd:YAG laser have not yet been studied. The salient issue is how to handle the thermally induced birefringence of the laser medium and the electro-optical crystals[14,15], especially in quasi-three-level lasers.
In our work, we observed depolarization phenomenon in the RTP electro-optic modulators (where no voltage was applied) in a quasi-three-level laser with a conventional resonator configuration. The severe depolarization loss made it unable for us to obtain a -switching laser. Our approach to this problem was to utilize a quarter-wave plate (QWP) behind the double-crystal RTP Pockel’s cell, as well as a composite laser medium with a low-concentration dopant. Using this type of resonator, we were able to obtain an E-O -switched 946 nm Nd:YAG laser at 1 kHz. Under an incident pump power of 11.1 W, a maximum output power of 350 mW at 1 kHz repetition frequency and 11 ns pulse duration was achieved with an output coupler of 10% transmission . The corresponding peak power was as high as 32 kW.
In our work, the RTP crystal was selected as a Pockel’s cell since it has a low half-wave voltage and can operate at relatively high repetition rates[16]. In order to compensate for the natural birefringence effect of the RTP crystal, caused by temperature variation, a double-crystal temperature compensation scheme was employed as an electro-optic modulator[17]. As shown in Fig. 1(a), the pump source was a 0.22 numerical aperture, 400 μm core diameter fiber-coupled laser diode, emitting at 808 nm. Pump pulses were 200 μs long at 1 kHz. A 2:3 lens-coupling system was employed in order to image the fiber end into the laser crystal. The gain element was an 11 mm long bonding YAG slab with a 5 mm long 0.5% Nd-doped portion in the middle, and two 3 mm long un-doped end caps. Both optical faces of the crystal had an anti-reflex coating for the laser wavelength at 946 nm, for the pump wavelength at 808 and at 1064 nm, to avoid parasitic lasing at the strongest transition of the ion. A convex–concave resonator was used in the work that had a length of 100 mm, with an input mirror of radius and an output mirror of 125 mm radius. The resonator was chosen to provide some compensation for the thermally induced lens within the laser crystal[18]. Electro-optical -switching requires a well-defined state of polarization of the radiation, which can be achieved by placing a polarizer in the cavity. The linearly polarized output power at 946 nm was measured to be 0.082, 0.606, and 1.415 W, at pump powers of 3.6, 7.4, and 11.1 W, respectively.
Sign up for Chinese Optics Letters TOC. Get the latest issue of Chinese Optics Letters delivered right to you!Sign up now
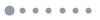
Figure 1.Experiments of thermal birefringence in electro-optic crystal (no voltage is applied); (a) conventional resonator; (b) conpensated resonator.
However, a rapid decrease and vanishing of output power was observed after inserting the double-crystal RTP into the linear resonator emitting polarized light at 946 nm. The hypothesis is that the depolarization loss caused by the double-crystal RTP was too high, and led to the laser’s inability to oscillate. Thus, it would not have been possible to operate a quasi-three-level 946 nm laser employing the conventional configuration in an E-O -switched mode at 1 kHz [see Fig. 1(a)]. In order to clarify the nature of the depolarization effect that appears in the double-crystal RTP, the red light (635 nm, 5 mW) emitted by the semiconductor laser impinged upon the resonator output mirror (the radius of curvature, ). After passing through the double-crystal RTP, the red light was deflected out of the resonator at the polarizer, as shown in Fig. 1(a). Results obtained are shown in Fig. 2(a).
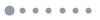
Figure 2.Beam patterns of semiconductor laser; (a) with noncompensation; (b) with compensation.
Obvious deformation of the beam shape, which becomes more severe with the increase of the pump power, can be observed. We attribute this to the nonuniform index in the double-crystal RTP due to weak light absorption. The spatial distribution of the laser beam, which is less uniform in the quasi-three-level laser and surface cooling of the electro-optic crystal, produces a temperature distribution within the crystal. Reference [19], for instance, explained that when the resonator is open (no voltage applied to the electro-optic crystal) the depolarized portion of the radiation leaves the cavity at the polarizer as a loss. They presented a compensated resonator by the use of two identical Pockels cells and a polarization rotator between the cells. In practice, this approach increases the cavity length, which is not beneficial for use in quasi-three-level lasers due to shorter thermal focal lengths compared with four-level lasers.
A simple compensation method was adopted in our work. Only one was placed between the double-crystal RTP and the output coupler [see Fig. 1(b)], with one optical axis parallel to the laser polarization. The idea of using this approach first appeared in Ref. [20], where the QWP was located between the input mirror and the laser rods to compensate the thermal birefringence in the active medium. Referring to this idea, in our work we changed the location of the QWP to compensate for the thermal birefringence in the electro-optical crystal.
As observed from Fig. 2(b), the beam pattern improves and the output power can be stabilized at a range after compensating. A comparison of the beam patterns with noncompensation and compensation clearly reveals the dramatic improvement in beam uniformity that results from the use of the birefringence compensation. The shape of the compensated output beam is not perfectly circular, and is hypothesized to be due to the incompletion of the compensation. However, this technique can provide a major reduction in depolarization loss, and makes it possible to realize the E-O -switched 946 nm Nd:YAG laser at a relatively high repetition rate. One caveat is to ensure that the 946 nm light beam of the long-pulse Nd:YAG laser and the red light are not conlinear, to avoid damaging the semiconductor laser.
Using the same pump conditions as before, the laser characteristics were measured in a free-running mode of operation in a cavity without , , or an electro-optic modulator in the output coupler transmittance, as shown in Fig. 3. The QWP between the double-crystal RTP and the output coupler () is the same as in Fig. 1(b). The other QWP between the polarizer and the double-crystal RTP () was used for on -switching, with one optical axis perpendicular to the laser polarization. Two different transmissions of the output coupler ( and 20% at 946 nm) were investigated. Figure 4 shows the results obtained in a quasi-continuous-wave pumping regime, with a repetition rate of 1 kHz and 200 μs pulse width. The maximum long-pulse output power of 1.825 W (with polarizer) and 1.415 W (without polarizer) were obtained at an output coupling of . Thus, the same output coupler transmission was used in the -switched operation.
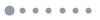
Figure 3.E-O -switched Nd:YAG laser resonator design for reducing the depolarization loss.
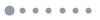
Figure 4.Long-pulse output power of Nd:YAG at 946 nm versus the incident pump power with different output couplers.
Since it would not have been possible to operate a quasi-three-level 946 nm laser by employing the conventional configuration [see Fig. 1(a)] in an E-O -switched mode at 1 kHz, as mentioned previously, the E-O -switched experiment was investigated in the compensated resonator. The average output power and pulse width related to the incident pump power are presented in Fig. 5. This shows a dramatic monotonous decrease in pulse durations from 43 to 11 ns, and an increase in average output power from 42 to 350 mW, with respect to incident pump power from 3.62 to 11.1 W. Figure 6(a) depicts the pulse train with a repetition rate of 1 kHz. The typical single-pulse envelope at maximum pump power of 11.1 W, as also shown in Fig. 6(b), corresponds to a pulse duration of 11 ns. For the maximum incident pump power of 11.1 W, the highest average output power of 350 mW was measured corresponding to an optical-to-optical conversion efficiency of 3.16%. The shortest pulses of the 11 ns FWHM length, and the almost 32 kW peak power were achieved for the 1 kHz repetition rate.
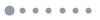
Figure 5.Average output power and pulse width as a function of incident pump power.
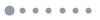
Figure 6.(a) Experimental pulse train at 11.1 W of pump power; (b) temporal shape of a single pulse.
In our compensation designs, the highest average power achievable is limited by the onset of damage to the QWPs ( and ) on which the small beam is incident. Other possible limitations at highest average output power arises due to the heating effects in the double-crystal RTP. However, the method described in this Letter for compensating thermally induced birefringence in the double-crystal RTP provides a configuration for an E-O -switched 946 nm Nd:YAG laser, at a relatively high repetition rate.
In conclusion, we demonstrate that the thermally induced depolarization in a double-crystal RTP will take place in a quasi-three-level 946 nm Nd:YAG laser. The depolarization loss makes the laser unable to oscillate by employing the conventional configuration. A resonator that compensated for the thermal depolarization is proposed, which makes it possible to realize an E-O -switched 946 nm Nd:YAG laser at 1 kHz. A maximum output power of 350 mW and a pulse duration of 11 ns are generated at a 1 kHz repetition rate, corresponding to an incident pump power of 11.1 W. The peak power of the -switched laser is . Despite the fact that compensation is not complete, this design can provide a major reduction in depolarization loss, and makes it possible to realize an E-O -switched 946 nm Nd:YAG laser at 1 kHz with a shorter cavity length and pulse width.
References
[1] P. Zeller, P. Peuser. Opt. Lett., 25, 34(2000).
[2] Y. Chen, H. Peng, W. Hou, Q. Peng, A. Geng, L. Guo, D. Cui, Z. Xu. Appl. Phys. B, 83, 241(2006).
[3] R. P. Yan, X. Yu, Y. F. Ma, X. D. Li, D. Y. Chen, J. H. Yu. Opt. Commun., 285, 4462(2012).
[4] F. Chen, X. Yu, R. P. Yan, X. D. Li, D. J. Li, G. L. Yang, J. J. Xie, J. Guo. Laser Phys., 23, 055002(2013).
[5] S. J. Yoon, J. I. Mackenzie. Opt. Express, 22, 8069(2014).
[6] H. Y. Zhu, C. W. Xu, J. Zhang, D. Y. Tang, D. W. Luo, Y. M. Duan. Laser Phys. Lett., 10, 075802(2013).
[7] M. Castaing, E. Hérault, F. Balembois, P. Georges, C. Varona, P. Loiseau, G. Aka. Opt. Lett., 32, 799(2007).
[8] R. Zhou, E. B. Li, H. F. Li, P. Wang, J. Q. Yao. Opt. Lett., 31, 1869(2006).
[9] L. Han, B. Yao, X. Duan, S. Li, T. Dai, Y. Ju, Y. Wang. Chin. Opt. Lett., 12, 081401(2014).
[10] B. Yao, X. Liu, X. Yu, X. Duan, Y. Ju, Y. Wang. Chin. Opt. Lett., 11, 031405(2013).
[11] R. Yan, X. Yu, X. Li, D. Chen, J. Yu. Appl. Phys. B, 108, 591(2012).
[12] Y. Lutz, D. Rytz, C. Gaudillat. Appl. Phys. B, 70, 479(2000).
[13] E. Nava, C. Mariottini, M. Tobia, E. Stucchi. Proceedings of the Sixth International Conference on Space Optics, 134(2006).
[14] I. P. Khristov, I. V. Tomov, S. M. Saltiel. Opt. Quantum Electron., 15, 289(1983).
[15] C. A. Ebbers, S. P. Velsko. Appl. Phys. Lett., 67, 593(1995).
[16] M. Roth, M. Tseitlin, N. Angert. Glass Phys. Chem., 31, 86(2005).
[17] D. Nickel, C. Stolzenburg, A. Beyertt, A. Giesen. Rev. Sci. Instrum., 76, 033111(2005).
[18] V. Magni. Appl. Opt., 25, 107(1986).
[19] S. Z. Kurtev, O. E. Denchev, S. D. Savoy. Appl. Opt., 32, 278(1993).
[20] W. A. Clarkson, N. S. Felgate, D. C. Hanna. Opt. Lett., 24, 820(1999).