Fig. 1. Schematics of nonlinear processes. (
a) Saturable absorption. (
b) Optical limiting
1.
Fig. 2. Experimental setups of Z-scan. (
a) Open aperture and close aperture Z-scan systems. (
b) Components to construct the open aperture Z-scan with tunable incident laser power
62.
Fig. 3. Experimental setups. (a) Laser ablation inside a vacuum chamber for selected gas matrix. (b) Laser ablation with the size selection of nanoparticle synthesis in air.
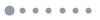
Fig. 4. Nanoparticles synthesized by laser ablation. (
a) TEM image of carbon nanoparticles synthesized with an average size of 6.5 nm. (
b) TEM image of carbon nanoparticles fabricated in liquid. (
c) TEM image of nano-diamond particles fabricated by laser ablation. The diamond nanoparticle was measured to have a grain size ~300 nm. (
d) TEM image of organic particles fabricated in water. (
e) SEM image of the Co nanoparticles synthesized. (
f) Optical images of various metallic nanoparticles’ dispersions. Colors of Au, Ag, Al, Cu, Ti, and Ni nanoparticle dispersions are dark red, orange, transparent, dark green, blue, and dark yellow, respectively. (
g) SEM image of CuO nanospindles. (
h) SEM image of silicon nanoparticles synthesized in water. Most nanoparticles were around 100 to 300 nm. Some were below 100 nm. (Inset: zoom-in SEM image). (
i) SEM image of TiO
2/Ag hybrid nanoparticles. The size ranged from 20 to 30 nm. (
j) SEM picture of GeO
2 nano-rods synthesized in water. (
k) SEM picture of the urchin-like ZnSnO
3 nanoparticles. Inset: zoom-in picture of a single urchin-like nanoparticle
58, 62, 73, 74, 120-122. Figure reproduced from: (b) ref.
74, American Institute of Physics; (c) ref.
120, Springer-Verlag; (d) ref.
121, Elsevier; (e) ref.
122, Elsevier; (h) ref.
62, under a Creative Commons Attribution 3.0 License.
Fig. 5. Optical nonlinear characterization for (
a) carbon nanoparticles, (
b) metallic nanoparticles, (
c) open aperture and (
d) close aperture Z-scan results for dielectric nanoparticles
62,75, 123. Figure reproduced from: (a) ref.
75, IOP Publishing; (b) ref.
123, SPIE; (c) ref.
62, under a Creative Commons Attribution 3.0 Licens.
Fig. 6. Hybrid nonlinear optical systems based on nanoparticles. (
a) Schematic diagram to demonstrate the GO system hybridized with transparent microspheres. The photonic nanojet can cause the enhancement of optical nonlinearity. (
b) and (
c) Nonlinear optical characterization and optical images of the reference group of pure GO and the GO+microspheres sample. Figure reproduced with permission from ref.
124, Optical Society of America.
Fig. 7. Dynamic nonlinear optical systems based on nanoparticles. (
a) Schematic to demonstrate GO+microsphere system in the frozen matrix. Local field enhancement improves the optical nonlinearity of GO. (
b) Nonlinear optical characterization to demonstrate the saturable absorption performance. (
c) SEM image of microspheres mixed inside the frozen matrix. (
d) Steps to show the preparation process to mix the GO and microspheres in the frozen matrix. (
e) Nonlinear optical performance of the phase change system under incident light at different intensities. Figure reproduced with permission from ref.
125, The Royal Society of Chemistry.
Fig. 8. Nonlinear optical systems based on solid-state phase change material. (
a) Nonlinear optical performance of phase change system under low laser fluence. Saturable absorption of silicon nanoparticles plays a dominant role. (
b) Performance of phase change system under high laser fluence. Nonlinear scattering induced by the formation of the micro-bubbles plays a dominant role. (
c) Z-scan characterizations on the tunable nonlinear optical performance of the phase change system. Figure reproduced with permission from ref.
72, Optical Society of America.
No. | Liquid | Material | Note | 1 | deionized water | Au59, Co57, Ag60, Ti61, Al61, Si62, ZnO63, Quantum dots (CdTe, CdSe, ZnTe)64,65, Carbon-based materials66,
Oxo(phthalocyaninato)vanadium67,
Alloys68 | Most popular liquid matrix | 2 | n-hexane, diethyl ether, toluene, 2-propanol, acetone, and methanol | Au57, Co57, Alloys69 | Relatively stable organic matrices | 3 | ethanol | Al70, Ti61, ZnO63 | Al slowly reacts with oxygen70 | 4 | chloroform, carbon tetrachloride, dichloromethane, cyclohexane | Al71 | Organic matrices with different polarities and densities | 5 | wax | Si72 | Phase change matrix |
|
Table 1. Common liquid matrices for laser ablation.
Table 2. Nanoparticles’ size comparison.