Abstract
Organic photoresponsive materials can undergo various reversible variations in certain physical and chemical properties, such as optical properties, electrochemical properties, conformation, and conductivity, upon photoirradiation. They have been widely applied in various optoelectronic fields, especially in information storage. We summarize research progress on organic photoresponsive materials for information storage. First, the design strategies and photoswitching mechanisms for various kinds of organic photoresponsive materials, including small organic molecules, metal complexes, polymers, supramolecules, and cholesteric liquid crystals, are systematically summarized. These materials exhibit reversible changes of absorption and/or emission properties in response to different wavelengths of light. Subsequently, the applications of these organic materials in information storage, such as data (re)writing and erasing, encryption and decryption, and anticounterfeiting, are introduced in detail. Finally, the current challenges and future directions in this rapidly growing research field are discussed. The review will provide important guidance on the future works about the design of excellent organic photoresponsive materials for optoelectronic applications.1 Introduction
In the current era of “big data,” the rapid growth in the amount of data generated each year has created more demand for ultracapacity storage media.1,2 At present, there are three mainstream modern information storage technologies, i.e., optical-, magnetic-, and semiconductor-based ones. Among them, optical information storage exhibits the merits of low cost, fast speed, long lifetime, easy portability, high storage density, and low power consumption, becoming an important information storage technology and being widely applied in many fields, including daily life, economy, military, etc.3–6 Photoresponsive materials can well realize information recording and readout because they can undergo a series of reversible changes in certain physical and chemical properties in response to light stimulus, which are specifically manifested as variations in optical properties (absorption and emission), solubility, viscosity, conformation, polarity, electrochemical properties, conductivity, refractive index, etc.7,8 Among these variations, optical properties play a decisive role in information storage because the success of information (re)writing and erasing, encryption and decryption, and anti-counterfeiting is directly judged by the change in the color and/or luminescent color of photoresponsive materials. Compared with solvent- or vapor-, thermal-, electro-, magnetic-, and mechano-responsive counterparts, photoresponsive materials are easier to realize optical information storage because light has many unique advantages including easy accessibility, no need of physical contact, high spatial and temporal resolution, and easy tuning of emission wavelength and intensity, showing great convenience in information storage in a noninvasive manner.9–12 On the one hand, the high spatial and temporal resolution enables photoswitching to be performed quickly and accurately, which ensures the smooth realization of information storage.10 On the other hand, light can precisely modulate the color and/or luminescence color of photoresponsive materials,7–9 thereby preventing information from being stolen or imitated. In general, photoresponsive materials can be reversibly interconverted between two or more discrete states with obvious different optical outputs through alternating light irradiation, realizing the activation or erasure of encoded information.13 Compared with the inorganic counterparts, organic photoresponsive materials exhibit unique advantages in light weight, low cost, good scalability, high flexibility, and compatibility with large-area solution-processing techniques including inkjet printing and screen printing.14–19 More importantly, their storage characteristics can be easily adjusted through molecular design-cum-synthesis strategies.14–17 Therefore, various organic photoresponsive materials have been successfully applied to information storage and have become promising candidates for single-molecule information storage. Their photoswitching characteristics usually depend on light-triggered isomerization of photochromic and/or photofluorochromic units such as ring opening-closure reaction and/or cis-trans isomerization, which result in variations at the molecular and macroscopic scale.7–10,16,20,21
Photochromic materials were first discovered by Fritzsche in 1867, and the term “photochromism” was put forward by Y. Hirchberg in the 1950s, meaning light and color.7–9,22 Photochromism refers to the reversible color changes of a photochrome between two isomeric forms by alternating light irradiation.7–9,21–24 Different wavelengths of light correspond to different colors, thereby realizing information recording, erasing, and rewriting. According to their relative thermal stability of the generated isomers, photochromic compounds are classified into P-type (photochemical) and T-type (thermal) ones.9,23,25–27 P-type photochromic molecules are thermally stable in two isomeric forms and require light irradiation to recover, while T-type photochromic ones are thermally unstable and spontaneously return to their original state within milliseconds to a few minutes. For practical information storage applications, photochromic materials have high sensitivity, fast response time, nondestructive readout capabilities, and excellent fatigue resistance.28,29 Representative organic photochromic molecular parents for information storage include diarylethene (DAE), spiropyran (SP), and azobenzene (Azo) (Fig. 1). In accordance with the above description, DAE belongs to P-type photochromic molecules, while SP and Azo are T-type ones. Through the reasonable modification and expansion of the three molecular parents mentioned above, organic photochromic materials for information storage have been extensively designed and developed. The current research on organic photochromic information storage materials mainly focuses on small organic molecules, metal complexes, and cholesteric liquid crystals (CLCs), which are mainly based on the reversible photoisomerization of photochromic units.
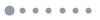
Figure 1.Photochromic molecules encompassed in this review and their photoisomerization processes: (a) diarylethene, (b) spiropyran, and (c) azobenzene.
Organic photofluorochromic information storage materials have been extensively explored for 20 years,9 drawing particular interest owing to their widespread application in information security protection. These materials can achieve high-level security encryption and anticounterfeiting applications because they have more information processing methods than photochromic materials. Photofluorochromism involves the reversible fluorescent color change of a photochrome under alternating light irradiation. Different wavelengths of light output correspond to different fluorescent colors, which shows great convenience in information encryption, decryption, and anticounterfeiting. The approaches to designing organic photofluorochromic materials for information storage are mainly based on small organic molecules, polymers, and supramolecules. The main strategies for designing small organic photoswitching fluorophores include the following aspects: (1) designing fluorophores with photoisomerization properties; (2) designing fluorophores with aggregation-induced (enhanced) emission (AIE/AIEE) characteristics; (3) introducing a photochromic group and a fluorophore that can produce Förster resonance energy transfer (FRET) effect between them. Organic photoswitchable fluorescent polymers for information storage are mainly concentrated on the design of nonconjugated polymers and polymer nanomaterials. Nonconjugated polymers are usually formed by introducing photochromic units and fluorophores to nonconjugated backbones, and their photoswitching characteristics can be fine-tuned by controlling the ratio of different monomer units. As for polymer nanomaterials, one photochromic molecule and one or more fluorophores are combined together through one-pot microemulsion polymerization to flexibly adjust the emission colors by varying these dyes and their combinational ratios.30–32 The molecules are fixed in a polymer matrix so as to isolate each other or the environment to prevent undesirable energy transfer or aggregation caused quenching.32–34 The reversible photoisomerization of different photochromic monomers is responsible for the reversible color changes of these polymers, while the fluorescence photoswitching behaviors are mainly ascribed to the reversible FRET on-off switching from fluorophore to photochromic unit, or between different fluorophores. Supramolecules are designed and synthesized by dynamically regulating the noncovalent host–guest interaction between fluorophores and photochromic molecules, or assembling photochromic or photofluorochromic units into supramolecular polymers. Furthermore, supramolecules, as the dynamic platforms for multicomponent organization, are conducive to the realization of cascade photoinduced energy and/or electron transfer processes.26,35 Similar to polymers, their photoswitching behaviors are also attributed to the photoisomerization of the photochromic units and the FRET effect from the fluorescent donor to the photochromic guest or between different fluorophores.
Sign up for Advanced Photonics TOC. Get the latest issue of Advanced Photonics delivered right to you!Sign up now
To date, several reviews on photochromic and photofluorochromic materials have been reported in detail.21–27,36–47 However, there is no relevant systematic summary on their application in information storage. In view of the rapid development of this field, it is very necessary to systematically summarize and discuss the current research progress and point out the future development of organic photoresponsive materials for information storage. In this review, we summarize and comment on the recent research progress of organic photoresponsive materials for information storage that includes small organic molecules, metal complexes, polymers, supramolecules, and CLCs. Their photoswitching mechanisms, design strategies, and information storage application are introduced in detail. Finally, the future challenges and opportunities in this field are illuminated.
2 Photoswitching Mechanisms
Photochromic and photofluorochromic molecules are key components to realize optical information storage. Specifically, optical information storage depends on the reversible changes in absorption and/or emission properties between two isomeric forms of photochromic and/or photofluorochromic units upon alternating photoirradiation. In general, their photoswitching characteristics can be achieved by tuning the isomeric conversion, energy transfer process, and noncovalent interaction.
Isomeric conversions are mainly aimed at small organic photochromic molecules and other organic materials containing photochromic groups for information storage. The photochromic molecules involved in this review are DAE, SP, and Azo (Fig. 1). Reversible pericyclic reaction (i.e., DE and SP) or cis-trans isomerization (i.e., Azo) is conducted by alternating ultraviolet (UV) and visible light irradiation or heat treatment, accompanied by obvious variations in color, thereby realizing information recording, erasing, and rewriting. DAEs were first reported by Mohri and Irie in 1988.48 They can transform into the colored ring-closed forms from the colorless ring-open forms under UV irradiation [Fig. 1(a)].21–27,40–42,44–46,49 The recovery process is thermally stable and driven only by visible light irradiation. The remarkable properties allow their successful application in information storage, including rapid light response, good reversibility, outstanding fatigue resistance, excellent thermal stability, and high photoisomerization quantum yield.50–55 SP also undergoes reversible ring opening-closure photoisomerization. It becomes the purple merocyanine (MC) form under UV light irradiation, while it reverts into the colorless SP form by heating or irradiating with visible light [Fig. 1(b)].44–47 Photosensitive Azo, a typical model of cis-trans isomerization, was first reported in 1937 and has been extensively studied in information storage because of its easy synthesis, highly efficient isomerization, fast photoresponsiveness, and high resistance to photobleaching.26,56,57 Under UV irradiation, its cis-trans isomerization can take place, and the reverse process can occur by irradiating with visible light or thermal relaxation [Fig. 1(c)].23,25,26,40
Interestingly, some photogenerated isomers have inherent fluorescent properties.9 Their fluorescence photoswitching can be easily implemented by reversible conversion between a nonemissive isomer and an emissive isomer under light irradiation or heat treatment.9 In addition, some fluorescent molecules that respond to photoirradiation can also achieve fluorescence switching. More generally, however, fluorescence photoswitching for information storage is usually achieved by introducing photochromic and fluorescent components into one system, i.e., small organic molecules, polymers, and supramolecules. In these systems, fluorescence can be turned on and off reversibly based on intramolecular FRET from the fluorescent moiety to the photochromic unit. Since the emission colors of different photochromes can be predicted, the final emission spectra can be modulated and expanded in the case that FRET is designed to occur.58–60 However, for most existing photofluorochromic information storage materials, only single-color fluorescence on/off photoswitching is realized based on the above mixture system.9 Multicolor fluorescence photoswitching for information storage can be obtained by mixing three or more fluorescent molecules into one system and using two-step (or multistep) FRET between them.35 However, there are few relevant reports due to the high matching requirements for absorption and emission spectra between fluorophores for information storage. If the introduced fluorescent component has an AIE/AIEE effect, a two-component system containing a photochromic group and a fluorescent unit can also achieve fluorescence photoswitching. In addition, noncovalent interaction, such as hydrogen bonding, between photochromes, or photochromes and other components can assist in the construction of photoresponsive information storage materials.
3 Small Organic Photoresponsive Molecules
Small organic photoresponsive molecules have been widely studied for information storage owing to their low cost, easy structural modification, and tunable photophysical properties.61 Among them, DAE and its derivatives have gained great attention owing to their rapid light response, good thermal stability, outstanding fatigue resistance, and high photoisomerization quantum yield.50–55 In addition, SP and its derivatives, photoresponsive tetraphenylethylene (TPE) derivatives, redox dyes, and dianthryl sulfoxides were also successfully applied to information storage.
3.1 Photoresponsive Diarylethene Derivatives
Kim and coworkers designed and synthesized three hydrophilic photochromic DAE derivatives 1 to 3 (Fig. S1 in the Supplemental Materials) as erasable ink materials for full-color ink-jet printing.62 Compounds 1 to 3 were highly soluble in water, and their colorless solutions were changed to cyan, magenta, and yellow, respectively, under UV light irradiation. The recovery process occurred upon visible light irradiation. The reversible photoswitching process is attributed to the reversible photoisomerization between the ring-opening forms and the ring-closure forms of these compounds. Text was printed onto A4 size paper with cyan ink (aqueous solution of compound 1) by ink-jet printing. The color intensity and resolution of the printed content are very suitable for regular reading, and its clarity lasted 3 h under ambient conditions. Moreover, the printed text was completely erased by visible light irradiation, and other new information can be reprinted on the same A4 size paper [Fig. 2(a)]. In addition, a full-color image was printed using an optimally mixed aqueous solution of compounds 1 to 3. Similarly, the image was erased under the irradiation of visible light, and a new erasable full-color image was reprinted on the same A4 size paper. In order to realize information storage on cellulose paper, Felpin and coworkers first proposed the concept of covalent printing, which utilized the spatially controlled thiol-yne ligations between the photochromic DAE derivative 4 or 5 (Fig. S1 in the Supplemental Materials) and the cellulose paper to create robust photochromic patterns.63 First, dithiodiglycolic acid was grafted onto cellulose paper (Cell-DiS). Second, Cell-DiS was inserted into the sample holder composed of two aluminum plates, in which the top plate was processed into the letter “U.” Finally, the letter “U” was printed on Cell-Dis with xenon lamp irradiation by immersing Cell-Dis into the DMSO solution containing compound 4 or 5 and a radical initiator [Fig. 2(b)]. The red letter “U” appeared and disappeared on the cellulose paper by alternating 254-nm and light irradiation, which is attributed to the reversible photoisomerization of compounds 4 and 5. Moreover, the robust covalent patterns are insensitive to successive washings compared to those created by the noncovalent physical adsorption process. This work provides a new strategy to build robust photochromic patterns on the cellulose paper, which makes it possible for anticounterfeiting on the cellulose paper.
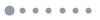
Figure 2.(a) Images about information print-erase cycles on the same A4 size paper by employing the aqueous solution of compound 1 as cyan ink. Reproduced with permission from Ref. 62, © 2016 WILEY-VCH Verlag GmbH & Co. KGaA, Weinheim. (b) General strategy for covalent printing of photochromic compounds 4 and 5 (DBE). Reproduced with permission from Ref. 63, © 2019 American Chemical Society. (c) Fluorescence photographs of PMA film containing compound 6 under the irradiation of 302 nm (erasing), 621 nm (writing), and 720 nm (reading). Reproduced with permission from Ref. 64, © 2019 American Chemical Society. (d) Photographs of the four different solutions and gel states of the mixed organogel. Reproduced with permission from Ref. 65, © 2017 WILEY-VCH Verlag GmbH & Co. KGaA, Weinheim.
Apart from the photochromic DAE derivatives, a series of DAE-based luminescent materials have also been extensively studied for information storage. A near-infrared (NIR) fluorescence photoswitching molecule 6 (Fig. S1 in the Supplemental Materials) was designed by connecting four photochromic DAEs on the bay positions of NIR fluorescent terrylenediimide moiety via oxygen bridges for erasable nonvolatile optical memories.64 The reversible red fluorescence and nonfluorescence photoswitching behavior was observed upon alternating 302/621-nm light irradiation because of the ring opening-closure photoisomerization of the DAEs moieties. The fluorescence quenching was mainly ascribed to the electron transfer from the ring-closure form DAEs to the excited terrylenediimide group under the irradiation of 302-nm light. The high fluorescence on/off ratio, decent reversibility, and fatigue resistance allowed the successful application of compound 6 in erasable nonvolatile optical memories. The memories were fabricated by spin-coating a chloroform solution containing compound 6 and polymethacrylate (PMA) onto quartz plates. The positive and negative patterns under fluorescence and bright field were repeatedly recorded and erased by utilizing the patterned illumination through different photomasks. An illuminated pattern was written under 621-nm light irradiation by a photomask, followed by the pretreatment of the spin-coated PMA films containing compound 6 with 302-nm light. The pattern was erased by irradiating 302-nm light, and a new pattern was rewritten with another photomask upon 621-nm light irradiation. Under 720-nm light irradiation, the recorded information was nondestructively read out because the reading wavelength was separated from the writing (621 nm) and erasing (302 nm) wavelengths [Fig. 2(c)]. Similarly, a fluorescent photoswitching molecule 7 (Fig. S1 in the Supplemental Materials) was designed and synthesized for the photorewritable fluorescent pattern, where three DAEs were connected to the bay positions of perylenemonoimide moiety via oxygen bridges.66 Compound 7 exhibited a large fluorescence on/off ratio and high fluorescence switching speed. Reversible fluorescence photoswitching behavior was also displayed upon alternated 302- and light irradiation. The fluorescence quenching was mainly attributed to the intramolecular FRET from the perylenemonoimide unit to the ring-closure form of DAEs by irradiation with 302-nm light. Photorewritable fluorescence patterning was displayed on PMA film doped with compound 7 by structured illumination. Positive and negative patterns can be written/erased/rewritten upon alternated 302- and light irradiation with the aid of a Cu-grid photomask. Liu et al. designed a photochromic DAE-bridged bispyridinium salt 8 (Fig. S1 in the Supplemental Materials) with AIE properties for photowritable and photoerasable applications.67 Due to the reversible ring-opening and ring-closure reactions of the DAE moiety, compound-8-embedded polymethyl methacrylate (PMMA) or polyvinylidene fluoride (PVDF) thin film exhibited reversible photochromism and fluorescence photoswitching under alternated irradiation with 254- and light. The colors of the PMMA and PVDF films containing compound 8 were altered between pale yellow and blue, and their corresponding fluorescence was changed between green and dark during the reversible photoswitchable process. Therefore, photowritable and photoerasable application was displayed using simple hollowed-out molds. Initially, the mold was covered on the compound-8-contained PMMA or PVDF films. Upon 254-nm light irradiation, the fluorescence outside the mold was quenched, and the part covered by the mold retained the original fluorescence. At this time, the desired pattern was clearly displayed on the PMMA or PVDF film after removing the mold. Subsequently, after irradiating the resulting film with light of , the printed pattern was erased because of the recovery of the quenched fluorescence. The new pattern can be reprinted on PMMA or PVDF film-containing compound 8, and the writing/erasing process can be repeated many times. Miao and coworkers developed a series of photochromic DAE derivatives 9 to 14 (Fig. S1 in the Supplemental Materials) by connecting aromatic substituent and TPE moiety through the DAE bridge.68 They showed typically AIE or AIEE characteristics, as well as photochromism under the irradiation with alternative UV and visible light. Among them, compound 10 loaded on PMMA film still exhibited photochromic behavior due to the reversible photoisomerization reaction of the DAE moiety. Hence, the rewritable information storage was achieved on the PMMA film loading with compound 10 by irradiating the photomask with the alternative UV and visible light. Similarly, Zhu and coworkers designed a photochromic fluorophore 15 (Fig. S1 in the Supplemental Materials) by introducing two TPE units into DAE moiety, which simultaneously exhibited AIE and reversible fluorescence photoswitching characteristics.69 Compound 15 in the solid state exhibited reversible photochromic and fluorescence photoswitching behaviors under alternated irradiation of UV and visible light. Color and emission switched between light yellow and blue purple, nonemission, and blue-green, respectively. Therefore, optical memory was achieved using fluorescence photoswitching. Information can be reversibly recorded (), erased (UV light), and read out (). In addition to the above single-color fluorescence photoswitching, Park and coworkers first developed a multistate switchable and multicolor adjustable organogel, which consisted of a cyanostilbene organogelator 16 (Fig. S1 in the Supplemental Materials) with AIEE properties and a photochromic DAE derivative 17 (Fig. S1 in the Supplemental Materials).65 Compound 16 had no emission in the solution, but displayed strong blue fluorescence in the gel state due to the AIEE characteristics, while the ring open form of compound 17 in both the solution and solid matrix had no emission, and its ring closed form displayed green fluorescence. Owing to the reversible solution and gel transformation of compound 16 under the alternated heating and cooling treatment, and the reversible ring-opening and ring-closure reactions of compound 17 under alternated UV and visible light irradiation, the mixed organogel was reversibly switched between four different states [nonemissive solution, green-emitting gel, blue-emitting gel, and green-emitting solution, as shown in Fig. 2(d)] by orthogonal stimulus of light and heat. The mixed solution was nonemissive. Under visible light irradiation, the mixed solution exhibited green fluorescence owing to the generation of the ring-closure substances of compound 17. Since the good thermal sensitivity of compound 16 and thermal stability of compound 17, the nonemissive solution and green-emitting solution became blue-emitting gel and green-emitting gel, respectively, by cooling treatment. The blue-emitting gel turned to the green-emitting gel under UV irradiation because of the energy transfer from the gel state of compound 16 to the ring-closed form of compound 17. The reverse processes were performed under alternating treatment of light and heating. Based on its excellent multistate switchable and multicolor adjustable characteristics, the mixed organogel in a quartz cell was applied in rewritable optical recording. The green fluorescent gel letters were written on the gel background with blue fluorescence by irradiating the mask with UV light. After removing the mask, the green fluorescent gel filled the entire quartz cell under further UV light irradiation and then turned into the green solution by heating. Subsequently, a nonemissive solution was obtained by irradiating the green fluorescent solution with visible light irradiation and then turned into a blue gel after cooling treatment. Next, new information recording and erasing processes can be implemented again. These materials realize information writing-erasing cycles on different substrates, especially on ordinary A4 paper and cellulose paper, which clearly shows the practical applicability of information storage.
3.2 Photoresponsive Spiropyran Derivatives
Among the photochromic compounds, spiropyran and its derivatives are also widely considered in information storage due to their remarkable photosensitivity, photoresponsiveness, photostability, and reversibility.70 In order to achieve the solid-state photoswitching of small organic molecules, Tian and coworkers designed and synthesized a photoresponsive compound 18 (Fig. S1 in the Supplemental Materials) by covalently linking two SP units to a highly sterically bulky AIE molecule 9,10-distyrylanthrance.71 Compound 18 exhibited reversible photochromism and fluorescence photoswitching under alternated UV and heat/visible light treatment. By irradiating its solid powder or thin film with 365-nm light, compound 18 and its fluorescent color immediately turned to dark purple from yellow and to red from yellow, respectively. Efficient photoswitching between compound 18 and its isomer was ascribed to the reversible photoisomerization between SP and MC moieties and the intramolecular energy transfer from 9,10-distyrylanthrance to the MC unit. Meanwhile, photoswitching was further promoted by the large free volumes of compound 18 caused by the nonplanar molecular structure of 9,10-distyrylanthrance moiety and the intramolecular hydrogen bonds between the 9,10-distyrylanthrance and MC units. Anticounterfeiting application was demonstrated using compound 18 as an anticounterfeiting ink. A pale yellow pattern was displayed by printing an umbrella pattern on the filter paper with the compound 18 solution and completely drying under ambient conditions. The color of the umbrella pattern turned to blue violet under 365-nm light irradiation and returned to the original state by heating at 90°C for 1 min. The corresponding fluorescence changed reversibly between bright yellow and red. Hirai and coworkers developed a spiropyran-cholesterol conjugated organogelator 19 (Fig. S1 in the Supplemental Materials) for information storage.72 Compound 19 formed a yellow gel in benzyl alcohol at room temperature and turned into a homogeneous purple solution at . The purple gel was produced by cooling the purple solution to 25°C. Owing to the formation of hydrogen bonding between the MC form and benzyl alcohol, the purple solution cannot form a colorless solution or gel under visible light irradiation or heating treatment. However, purple gel and yellow gel can be mutually transformed under alternated UV and visible light irradiation due to the reversible photoisomerization between the colorless SP form and purple MC form. Hence, writable/erasable information storage was realized using the gel as active material. The purple solution was introduced into the quartz cell to form a purple gel. Subsequently, the pattern was presented on the quartz cell by irradiating the photomask with visible light for 3 min. After removing the photomask, the information can be erased by irradiating UV light. New information can be rewritten and erased using a new photomask. At present, there are few studies on spiropyran-based photoresponsive information storage materials, which may be caused by their poor photoswitching cycles on a solid state or solid matrix. Therefore, new strategies should be explored to design more excellent SP materials for information storage.
3.3 Photoresponsive Tetraphenylethylene Derivatives
TPE, a common AIE material, is mostly nonfluorescent in the solution state but fluorescent in the aggregate/solid state due to the limitation of intramolecular rotation.73–76 This characteristic is very suitable for photoresponsive information storage. In addition to the above DAE-TPE counterparts, another two photochromic fluorophores 20 and 21 (Fig. S1 in the Supplemental Materials) were designed and synthesized for anticounterfeiting by combining the TPE unit with photochromic moiety naphthopyran or spirooxazine.77 They all show significant photochromic and AIE characteristics, and reversible solid-state color and luminescence switching were displayed under the alternating treatment of UV and visible light, which result from the photoisomerization between the open-ring and closed-ring forms of naphthopyran unit and the energy transfer from TPE moiety to the ring-opening form of naphthopyran unit. Taking compound 20 as an example, under visible light irradiation, its yellow turned to brown, and its blue fluorescence was quenched. The initial state was restored under UV light irradiation. Therefore, photoswitchable patterns were demonstrated using a photomask and compound 20 solution as anticounterfeiting ink. Brown patterns with nonemission were written on the TLC plate by irradiating the photomask with UV light for 40 s. The printed pattern disappeared under ambient conditions for 20 s, and new information can be rewritten. A cage compound 22 (Fig. S1 in the Supplemental Materials) was designed and synthesized for photopatterning and anticounterfeiting applications, in which AIE-active TPE derivative 23 (Fig. S1 in the Supplemental Materials) acted as fluorophore, and the 2-nitrophenyl group served as a quencher.78 Compound 22 was nonfluorescent in both the solution and aggregated state owing to the photoinduced electron transfer (PeT) process from compound 23 to the 2-nitrophenyl group. However, by irradiating its aggregated state with UV light, the 2-nitrobenzyl group on compound 22 was cleaved, and the strong cyan fluorescence from product 23 could be observed. Therefore, photopatterning and anticounterfeiting applications were realized by photomask technology. First, the filter paper was soaked with the THF solution of compound 22 and dried by compressed air. The mask was then placed on the treated filter paper. After irradiation with UV light, the desired pattern appeared on the filter paper. Subsequently, the pattern can be erased by removing the mask and irradiating the filter paper with UV light. At this time, the fluorescence of the mask part was activated, and the entire filter paper showed cyan fluorescence, so the pattern disappeared. Tang and coworkers developed a TPE derivative 24 with AIE properties, multistate mechanochromism, and self-recovery photochromic fluorescence for multidimensional anticounterfeiting guarantees.79 Mechanochromism refers to the reversible changes in color and/or emission colors of a material in response to external forces.76,79 The original powder 24 (Fig. S1 in the Supplemental Materials) exhibited a bright cyan emission, and the emission remained unchanged after grinding. The ground powder 24G with cyan emission turned into blue-emissive powder 24F after being exposed to dichloromethane for about 10 s, and the reverse process was easily achieved by gentle grinding. Powder 24H with dim fluorescence was obtained by annealing powders 24G or 24F at 120°C for 10 s, and the reverse processes were implemented by the grinding and smoking of powder 24H, respectively. The four powders are white when viewed with the naked eye under natural light, which is beneficial to their application in anticounterfeiting. Interestingly, the white powder 24H or transparent crystal 24C turned deep red upon UV light irradiation for , and the red color faded within 60 s after removing the UV light. Mechanochromism and photochromism were attributed to the weak intermolecular interactions caused by the head-to-tail stacking of the molecules and the formation of photocyclization intermediates under UV light irradiation, respectively. The multidimensional anticounterfeiting application was demonstrated based on the excellent mechanochromism and photochromism of compound 24 [Fig. 3(a)]. The pattern with multiple color combinations was designed on the exercise banknote. First, the background with blue emission was prepared by fuming the ground powder 24G. Second, the rose shape was embroidered by heating the middle region of the blue-emissive background. Finally, the contour of rose and leaves was drawn by grinding. Blue background, contour of rose, dim petals, and cyan leaves appeared after turning on the UV light. When increasing the UV light intensity, the rose petals turned red. The rose bloomed with a bright red color and gradually withered and disappeared after turning off the UV light. Similarly, different paintings or patterns can be designed depending on the specific requirements of personalized anticounterfeiting. To date, there are few researches on TPE-based photoresponsive materials.68,69,77–79 Reasonable designs utilizing their AIE characteristics will greatly enrich photoresponsive systems for information storage.
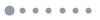
Figure 3.(a) Multidimensional anticounterfeiting demonstration based on compound 24. Reproduced with permission from Ref. 79, © 2019 WILEY-VCH Verlag GmbH & Co. KGaA, Weinheim. (b) Multilevel security information printing demonstration using zinc salts 40, 41, and 43 as the inks. Reproduced with permission from Ref. 80, © 2020 Science. (c) Information encryption and decryption demonstration by employing photoresponsive luminescent lanthanide-containing hydrogels 44 to 46. Reproduced with permission from Ref. 81, © 2019 WILEY-VCH Verlag GmbH & Co. KGaA, Weinheim. (d) Various patterns with different structural colors obtained using different photomasks. Reproduced with permission from Ref. 82, © 2020 WILEY-VCH Verlag GmbH & Co. KGaA, Weinheim.
3.4 Other Class of Small Organic Photoresponsive Molecules
In addition to the small organic photoresponsive molecules mentioned above, some photoresponsive redox dyes, single crystals, and dianthryl sulfoxides derivatives have been also developed for information storage. Yin and coworkers prepared a rewritable paper composed of a redox dye (methylene blue 25, Fig. S1 in the Supplemental Materials), titanium dioxide () nanocrystals, and hydroxyethyl cellulose.83 Dye 25 is blue in an oxidizing environment and transforms into its leuco form in a reducing environment. In this system, the oxidation and reduction of dye 25 were responsible for the basic conversion of printing and erasing. nanocrystals were used to photocatalyze the reduction reaction of dye 25 under UV irradiation. Hydroxyethyl cellulose can prevent the rapid spontaneous oxidation of colorless dye 25 in the presence of oxygen because hydrogen bonding was formed between groups on hydroxyethyl cellulose and groups on dye 25 and its leuco form. The 25/hydroxyethyl cellulose composite film exhibited excellent reversibility and repeatability, making it a rewritable paper for printing and erasing. Required information was printed on rewritable paper through a photomask under UV light irradiation, where the unexposed areas remained blue, while the exposed regions became white. Large-area printing is suitable, and the printed information can be still readable after 5 days under ambient conditions. Subsequently, the information was completely erased by heating the rewritable paper in the air at 115°C for . Moreover, the working colors of rewritable paper can be changed by replacing dye 25 with other photoresponsive commercial redox dyes, such as dyes 26 and 27 (Fig. S1 in the Supplemental Materials). This rewritable paper is expected to replace conventional paper to meet human daily use and environmental protection needs. Reddy and coworkers developed unique photochromic single crystals 28 (Fig. S1 in the Supplemental Materials) for rewritable information recording.84 The dark-orange single crystals 28 can be turned into their transparent polymer under visible light irradiation, while the reverse process could be performed via heating. Rewritable information recording was achieved by fabricating photopatternable filter papers and using the desired photomasks. The photopatternable filter papers were prepared by dip-coating the cellulose filter papers with the DCM solution () of the crystals 28 and then air-drying under dark conditions. Both whitish-yellow patterns with yellowish-orange background and dark-orange patterns with light-yellow background were constructed by covering the positive and negative photomasks on the dip-coated filter papers and irradiating them with 488-nm light for about 10 min. The dip-coated filter papers were changed to white within 30 min by exposing to 488-nm light and then returned into dark-orange by heating at 195°C for 1 to 2 min due to the reversible conversion between single crystals 28 and their polymers under alternating light and heat treatments. Other patterns can be rewritten using new photomasks. Furthermore, with a higher concentration (), the recorded patterns can be read up to the fifth day under room light and completely disappear by the seventh day. Interestingly, the photopatternable feature was also used for encoding hidden text with quick response (QR) codes, which can be decoded employing QR decoding software on smartphones. The encoded information was quickly erased by visible light within a few minutes and cannot be copied after erasure, thereby ensuring security. The duration of the information can be on-demand regulated by changing the monomer concentration for making patternable papers. Wolf and coworkers designed and synthesized three dianthryl sulfoxides derivatives 29 to 31 (Fig. S1 in the Supplemental Materials) that can produce red, green, and blue (RGB) fluorescence for advanced anticounterfeiting.85 Corresponding bianthracene photoproducts with RGB emission were produced by eliminating the SO bridge of compounds 29 to 31 upon light irradiation. The chromaticity coordinates of PMMA thin film containing three photochromes changed with the irradiation time and the irradiation wavelength. Therefore, the dynamic level of security was increased by controlling the light exposure time and/or excitation wavelength to obtain different colors and trajectories. Wong and coworkers first developed an excitation-wavelength-dependent triazole derivative for anticounterfeiting application.86 Under 254-nm light irradiation (high-energy excitation), the powder crystal 32 (Fig. S1 in the Supplemental Materials) exhibited bright yellow fluorescence as a result of the excited state intramolecular proton transfer process. However, owing to the influence of the excimers, its luminescence became light green after being irradiated with 365-nm light. At the same time, the photoluminescence quantum yield decreased from 16.5% to 3.9%. Encouraged by the excitation-wavelength-dependent characteristics, the anticounterfeiting ink was prepared by dispersing the crystalline powder 32 in ALOE VERA gel. The QR code was printed on the filter paper using the above ink and was invisible under natural light after the ALOE VERA gel was evaporated. After being excited with 254- and 365-nm light, bright orange and light green fluorescent QR codes were observed in the dark room, respectively, indicating this excitation-wavelength-dependent emission material can be applied in practical anticounterfeiting.
4 Photoresponsive Zinc(II) Complexes
Salicylaldehyde Schiff base has attracted considerable interests in information storage because of its simple synthesis, good fatigue resistance, and excellent photochromic performance.87 Hitherto, the metal complexes for information storage are mainly zinc(II) complexes that simultaneously contain spirolactam and salicylaldehyde Schiff base moieties. These zinc(II) complexes exhibit reversible photochromic behavior in the solution and solid matrix, which is very conducive to the realization of information storage. The spirolactam undergoes a ring-open reaction with a color change caused by the photoisomerization of the salicylaldehyde Schiff base unit from enol-form to keto-form upon UV irradiation.80,87–89 At this time, the zinc ion is complexed with the spirolactam-based ligand to reduce the negative charge density of the nitrogen atom, thereby stabilizing the ring-opening isomer.80,90,91 The reverse process is achieved through heating or darkness or visible light irradiation. Tang and coworkers developed a Zn(II) complex 33 (Fig. S1 in the Supplemental Materials) containing rhodamine B salicylaldehyde hydrazone for photoprinting.87 Complex 33 in the THF solution and solid state showed reversible photochromism in the dark and under UV light irradiation. Upon 365-nm light irradiation, the light yellow of complex 33 gradually changed to purple, and the emission reduced and converted into two weaker peaks. When in the dark, the light yellow emission with a strong peak was recovered. The photoprinting experiment was achieved by using complex 33 in poloxamer 407 (an ether polymer) solid. Letters were preorganized and recorded on the poloxamer 407 solid containing complex 33. Under UV light irradiation, letters were successfully displayed and then gradually disappeared until the next round of irradiation. Next, they designed and synthesized a photochromic rhodamine B salicylaldehyde hydrazone-based Zn(II) complex 34 (Fig. S1 in the Supplemental Materials) with a photoremovable o-nitrobenzyl group for photoactivatable self-hidden information storage.88 Complex 34 was photostable at wavelengths and yielded the complex 35-R (Fig. S1 in the Supplemental Materials) with red color and 2-nitrosobenzaldehyde under short-wavelength light () irradiation. After removing the light, the red 35-R returned to the colorless 35-L (Fig. S1 in the Supplemental Materials) in the dark, which was caused by the spirolactam ring-closed reaction. The information was input with UV light and disappeared completely after being placed in the dark for about 10 min. Upon the irradiation of 450-nm light, the information was read out successfully. More importantly, the information can be read multiple times because of the excellent fatigue resistance of complex 35. Finally, based on the same mechanism, three fluoran salicylaldehyde hydrazone Zn(II) complexes 36 to 38 (Fig. S1 in the Supplemental Materials) were also designed and synthesized for photopatterning applications.89 After UV light irradiation, the complexes 36 to 38 showed green, orange, and black in the solution and solid matrix, respectively, and displayed light yellow in the dark. Therefore, patterns successfully appeared in a thin layer of silica gel impregnated with these Zn(II) complexes after UV light irradiation. Moreover, the black two-dimensional barcode can also be constructed on a thin layer of silica gel containing complex 38. The high color contrast ratio before and after UV light irradiation made them easy to be identified. Inspired by the above works, Zhao and coworkers designed and synthesized a series of controllable photochromic Zn(II) complexes 39 to 43 (Fig. S1 in the Supplemental Materials) with different counterions for rewritable information recording and multilevel security printing.80 Complexes 39 to 43 exhibited photochromic behavior in low-polarity solvents and film, and their photochromic activity and coloration rate were increased significantly as the basicity of counter anions decreased. Their colors were changed from colorless to blue under UV light irradiation, and the original states were recovered under heating. These photoswitching behaviors were attributed to the reversible interaction and dissociation of the metal–ligand coordination bonds between and the spirolactam-based ligands, which were caused by the reversible photoisomerization reaction of the spirolactam-based ligand. Based on their excellent photochromic properties, rewritable information recording experiments were first conducted on the paper coated with polyethylene glycol-polypropylene glycol-polyethylene glycol (PEG-PPG-PEG) film containing complex 43. Various patterns were printed on the PEG-PPG-PEG film using photomask technology under UV light irradiation. After exposure to UV light for 2 min, the unexposed area remained colorless, while the exposed area turned to blue, thereby copying images from the photomask to the film. The patterns were completely erased by heating in air at 70°C for 1 min. Subsequently, multilevel security printing experiments were carried out on the newly made security paper that contains parchment as bottom layer, PEG-PPG-PEG film as the passivation layer, mixed spirolactam-based ligand and PEG-PPG-PEG film as the imaging layer, and another PEG-PPG-PEG film as the protection layer. Higher security information printing was achieved using , , and as inks [Fig. 3(b)]. The recorded information was invisible under ambient light and then gradually appeared by irradiating it with UV light for different times because complexes 40, 41, and 43 had different coloration rates under UV irradiation. The complete encrypted information can be accessed by the authorized personnel who knows the exact irradiation time and correct encryption algorithm, which greatly improves the security of hidden information.
5 Photoresponsive Polymers
Compared with small organic molecules and organometallic compounds, photoresponsive polymers are much more desired for information storage owing to their good film formation, excellent flexibility and scalability, 3D-stacking capability, and fast and facile accessibility, responsivity, reversibility, and controllability.70,92–96 Hitherto, nonconjugated polymers and polymer nanomaterials have been reported for information storage.
5.1 Photoresponsive Nonconjugated Polymers
Nonconjugated polymers are usually designed and synthesized by incorporating photochromic molecules and fluorophores to nonconjugated backbones, and their photoswitching properties are fine-tuned by controlling the ratio of different monomer units. Liao and coworkers introduced the SP moiety into the hydrogel by the host–guest interactions and photopolymerization for constructing photoswitchable patterns.97 The physical hydrogels were first produced by mixing the hosts (-cyclodextrin and α-cyclodextrin-SP) and the guest [poly(ethylene glycol) diacrylate (PEGDA)]. Subsequently, the covalently dual-crosslinked networks were formed by photopolymerization of the PEGDA. Owing to the photoisomerization of SP units, the dual-crosslinked hydrogel showed reversible photochromic behavior upon alternating UV and visible light irradiation. Hence, various red patterns were recorded on the hydrogel clearly through the photomask upon irradiation of UV light for 1 min and were erased after irradiation by visible light for 5 min. This excellent writing and erasing properties make SP-based hydrogels have great application prospects in information encryption and decryption. Next, they developed photoresponsive hydrogel actuators by introducing the SP unit into poly(N-isopropylacrylamide) for constructing various patterns.98 The hydrogel actuators showed reversible color and luminescence changes upon alternating UV and visible light irradiation owing to the reversible photoisomerization reaction between the colorless SP and colored MC. Specifically, pale yellow and no fluorescence were exhibited for hydrogel actuators under natural light, while the red and red fluorescence were displayed under UV light irradiation. The initial state was recovered after being exposed to visible light. Moreover, the hydrogel actuators showed different hydrophobicity/hydrophilicity for different solvents, i.e., self-rolling in ethanol and then stretching again in deionized water. Therefore, different patterns with a planar-to-3D shape were successfully constructed. A pattern was formed on the hydrogel film by a photomask under the irradiation of UV light for 2 min. After transferring the above hydrogel to ethanol for 2 min, the patterned hydrogel tube was formed on the outer surface. The pattern was erased after exposure in visible light for 1 min, and then the hydrogel film was stretched out by treatment with deionized water for 15 min. Next, new information can be drawn and erased reversibly on these hydrogel films by alternating UV and visible light irradiation. Lanthanide complexes have many intriguing optical properties including narrow emission bands, high luminescent efficiency, large Stokes shift, and long luminescence lifetime.81,99–101 Utilizing these excellent luminescence properties, Zhao and coworkers designed and synthesized photoswitchable lanthanide-containing hydrogels 44 to 46 (Fig. S2 in the Supplemental Materials) for information encryption and decryption.81 Hydrogels 44 to 46 are prepared by in situ copolymerization of acrylamide monomers with luminescent lanthanide centers and photochromic DAE derivatives. The yellow to red luminescence was fine-tuned by varying the molar ratio of and ions in the hydrogels. Reversible photoresponsive luminescence on-off behavior was displayed upon alternating UV and visible light irradiation owing to the reversible photoisomerization of DAE moieties. The FRET process from the lanthanide centers to the close form DAE was responsible for luminescence quenching under UV irradiation. At this time, the luminescence life and quantum yield were reduced sharply. For hydrogel 44, the luminescence lifetime and quantum yield changed from 872 to and from 12.3% to 0.6%, respectively, while these values of hydrogel 45 turned into 129 from and into 1.8% from 18.2%, respectively. Therefore, intelligent information encryption systems were constructed using hydrogel blocks containing no lanthanide complex monomers as background [Fig. 3(c)]. A luminescent array corresponding to code information was prepared by placing the red hydrogel 44, green hydrogel 45, yellow hydrogel 46, and nonluminescent hydrogel on the above background. Under daylight, the application software in the smartphone cannot recognize the information stored in the code. However, the luminescent code was easily recognized by a smartphone upon 254-nm light irradiation and disappeared under the irradiation with 300-nm light, realizing information encryption. The erased information can be decrypted and fully restored upon further exposure to visible light. New information encryption and decryption can be realized by altering the arrangement of the hydrogel blocks. This work offers a new way for the development of anticounterfeiting materials and devices.
In addition to hydrogels, some photoresponsive shape memory polymers have also been developed for information storage. Wang and coworkers incorporated SP molecules into the shape memory poly(ε-caprolactone) networks for high security information storage.102 The mixed polymer networks simultaneously exhibited reversible color and shape changes under the alternating stimulus of light or heat, which was attributed to the photoisomerization of SP moieties and the dynamic bond exchange of ester and urethane linkages. Hence, 3D anticounterfeiting labels were developed recognized by nonblind and blind. First, utilizing the dynamic bond exchange of ester and urethane linkages, the information recording process was conducted simultaneously in two steps. On the one hand, the Arabic numbers made of mixed polymers were embedded in the substrates made of poly(-caprolactone) networks. On the other hand, 3D embossed braille digital information was also successfully created on this substrate through molding technology. Afterward, both 2D digital information and 3D braille digital information were encrypted by pressing them with a smooth plate at 80°C and then fixing at 0°C. Finally, the 3D braille was decrypted through the shape memory recovery process by heating at 80°C, and its corresponding 2D information was decrypted by exposure to 365-nm light. Xu and coworkers designed and synthesized a shape memory polymer containing diselenide bond to create variable structural color patterning.82 The diselenide bond is a visible-light-responsive dynamic covalent bond, which can be formed, cleaved, and metathesized reversibly under certain conditions. The structural color was derived from the birefringence of stretched polymer, and its shape can be fixed while maintaining mechanical stress. However, the diselenide metathesis released the fixed stress under visible light irradiation. Single structural color patterns were designed with a photomask strategy and attached on the surface of the oriented material after fixing the pressure [Fig. 3(d)]. The masked patterns appeared after irradiating with 420-nm light for 15 min. The patterns were erased by partial or whole irradiation and rewritten again after uniaxial stretching. The tunable structural color patterns were realized by adjusting the irradiation time or wavelength of visible light with a commercial projector. Microareas with the same or different structural colors were realized by projecting single-color or multicolor patterns onto the oriented material. Besides the same irradiation time with different wavelength light, the multicolor structural patterns were also realized by irradiating different times with the same wavelength light. For example, rainbow flowers were displayed between two crossed polarizers by controlling the timing and order of each petal in the pattern. In the whole process, the optical signal was first stored as a mechanical signal and then was transmitted back to the optical signal for data storage. The new strategy for preparing multiple structural color patterns by a single visible light stimulus has many advantages, such as the simple and rapid production process for easy large-scale preparation, the high controllability of visible light in time and space with little damage to the material, and the difficult deformation for patterns, which has huge application potential in data storage, display devices, and anticounterfeit labels.
Based on the similar design strategy, other types of photoresponsive nonconjugated polymers have also been explored for information storage. Wang and coworkers first reported the photochromic organoboron-based copolymers 47 to 51 (Fig. S2 in the Supplemental Materials) for erasable writing and patterning by introducing photochromic boron compound on the side chains of the polymer with atom transfer free radical polymerization reaction.96 The random copolymers exhibited thermally reversible photochromism in the solution and solid state. The color turned into deep blue from colorless, and the fluorescence changed into deep blue from a bright sky blue after irradiating with 365-nm light owing to the reversible photoisomerization of boron units. The initial state was restored by heating at 120°C in a vacuum oven or 80°C in a hot water bath. Transparent polymer film was prepared by spin-casting the toluene solution of copolymer 51 onto a glass Petri dish and drying in the vacuum oven. A white pattern with bright sky-blue fluorescence was displayed using a photomask and irradiating with 365-nm light. It was then erased by heating at 120°C in a vacuum oven and rewritten upon 365-nm light irradiation. Moreover, information can be written on the glass surface using the above polymer solution as invisible ink. The writing was visible under 365-nm light irradiation and erased by immersing into the hot water. This work provides valuable guidance for the design and synthesis of organoboron-based information storage materials.
5.2 Photoresponsive Polymer Nanomaterials
In general, polymer nanomaterials are formed by combining one photochromic molecule with one or more fluorophores through one-pot microemulsion polymerization, and their photoswitching characteristics can be flexibly adjusted by changing these dyes and their combinational ratios.30–32 In order to obtain high-contrast red fluorescence materials, a donor 52 (Fig. S2 in the Supplemental Materials) with ultrahigh-brightness red emission and a photochromic acceptor 53 (Fig. S2 in the Supplemental Materials) were covalently linked to polymer nanoparticles by one-pot microemulsion polymerization.103 The nanoparticle was even times brighter than the quantum dots and exhibited reversible photoswitching in the solution, powder, and film. The color changed from red to gray, and the luminescence was quenched by UV light irradiation. The reverse process occurred upon visible light irradiation. The ring-opening to ring-closing reaction of acceptor 53 was responsible for the color change of the nanoparticles, and the FRET from the excited donor 52 to the ring-closure form of acceptor 53 caused the quenching of their strong red fluorescence. In addition, photoresponsive inks were prepared by mixing the nanoparticle and PMMA or PMA in chloroform to make patterns on a gravure mold. Upon alternating exposure to 365- and 625-nm light, the color and luminescence of the inks displayed the same reversible changes as that in the solution, powder, and film. Therefore, two types of nanoparticles (NP-1 without acceptor 53, NP-4 with acceptor 53) inks were used for anticounterfeiting and information encryption demonstrations. The Arabic numeral “8888” made by mixing NP-1 and NP-4 exhibited red and red emission under visible irradiation, while the gray and red fluorescent numbers “2019” appeared clearly under UV irradiation, which indicates that the nanoparticles have broad prospects in anticounterfeiting applications. In addition, the binary code was designed for information encryption demonstration using NP-1 and NP-4 as the “0” and “1” states, respectively. The initial information was displayed as “00000000.” Next, the information was decrypted (that is, the word “ED”) after UV irradiation for 20 s and was encrypted to the original state with visible irradiation. Dual-color photoswitchable fluorescent polymeric nanoparticles for rewritable patterning were first reported by introducing polymerizable BODIPY 54 (Fig. S2 in the Supplemental Materials) and photochromic molecule 55 (Fig. S2 in the Supplemental Materials) with a facile one-pot reversible addition-fragmentation chain transfer-mediated miniemulsion polymerization method.104 The polymeric nanoparticles displayed good hydrophilicity, high fluorescence contrast, excellent photostability, tunable energy transfer efficiency, and favorable photoreversibility. Reversible red and yellow-green fluorescence photoswitching was obtained under the alternative UV and visible light irradiation by tuning the FRET mechanism from BODIPY 54 to photochromic molecule 55. The red fluorescent patterns with yellow-green emission background and yellow-green fluorescent patterns with red emission background were prepared on filter paper using positive and negative photomasks with alternating UV and visible light irradiation. The switchable white-light generation has always been desired. It is easier to achieve switchable white-light generation with polymer nanoparticles than other material systems owing to their isolation effects and effective FRET process. Chen and coworkers developed a class of FRET-based single polymer nanoparticles for information encryption that can stably produce white light by embedding donor 56 (Fig. S2 in the Supplemental Materials) and acceptor 57 (Fig. S2 in the Supplemental Materials).32 Donor 56 has the AIE property, showing cyan emission in the aggregation state. The polymer nanoparticles also show cyan emission because there is no effective energy transfer between donor 56 and acceptor 57. Under UV irradiation, the FRET process was initiated by forming the ring-closure form of acceptor 57, while the emission of the nanoparticles was converted to red. White-light emission was obtained by the combination of the residual cyan emission from donor 56 and the red one from the ring-closure form of acceptor 57 in a rational design of the transfer ratio. The cyan, white, and red emissions can be reversibly converted by UV and visible light irradiation. Nanoparticles FPN-1, FPN-2, and FPN-3 containing donor 56 of different qualities were used to demonstrate the information encryption process. The nanoparticles showed cyan emission under visible light irradiation, but showed red (FPN-1), pink (FPN-2), and white (FPN-3) emission, respectively, after UV light irradiation. Therefore, the information stored in the 96-well plate was easily read by UV irradiation and was encrypted again by visible light irradiation. Based on this research work, Chen and coworkers also prepared a class of full-colored photoswitchable fluorescent polymeric nanoparticles toolbox including white light for information encryption [Fig. 4(a)]. A photochromic molecule 58 (Fig. S2 in the Supplemental Materials) and three primary fluorescent dyes 59 to 61 (Fig. S2 in the Supplemental Materials) were made into the reversible fluorescent photoswitchable nanoparticle toolbox by a simple one-pot miniemulsion method.60 The luminescence of the nanoparticles toolbox can be turned off by the FRET mechanism from the excited fluorescent dyes 59 to 61 to the closed-ring form of compound 58 under UV irradiation. The white-light to full-colored emission was achieved in both solution and film by the combination of the nonswitchable (, , containing 58) and switchable (, , containing 58) nanoparticles [Fig. 4(a)]. All of the mixture exhibited white light under visible light but shifted to various colors after UV irradiation [Fig. 4(a)]. Based on this, nanoparticles were successfully applied to information encryption using light as an encryption tool. The mixed samples with white fluorescent emission were filled into a 96-well plate, and the information was stored under visible light. Clear images with various luminescent colors were displayed after UV irradiation for 120 s. This work realized the complex fluorescence encryption, which showed their great potential in information storage and optoelectronic devices.
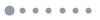
Figure 4.(a) Schematic illustrations of design strategy for full-colored photoswitchable fluorescent polymeric nanoparticles. Reproduced with permission from Ref. 60, © 2018 WILEY-VCH Verlag GmbH & Co. KGaA, Weinheim. (b) Full-color patterns realized using RGB Pdots as inks. Reproduced with permission from Ref. 105, © 2017 American Chemical Society. (c) Photopatterning and anticounterfeiting applications of SP-based latex nanoparticles. Reproduced with permission from Ref. 70, © 2018 American Chemical Society. (d) Schematic mechanism of the reversible dynamic regulating of the reflected wavelength of the light-driven chiral switches in CLC mixture. Reproduced with permission from Ref. 106, © 2010 Royal Society of Chemistry. (e) Reflection color images of the CLC mixture containing chiral switch 76 upon UV and visible light irradiation for different times. Reproduced with permission from Ref. 106, © 2010 Royal Society of Chemistry.
Apart from polymer nanoparticles, the FRET mechanism is also applied to design and develop fluorescent photoswitchable polymer dots and latex particles. Chan and coworkers designed and synthesized two categories of photoswitchable RGB polymer dots (Pdots) for anticounterfeiting applications by combining photochromic dyes 62 and 63 (Fig. S2 in the Supplemental Materials) with RGB semiconducting polymers 64 to 66 (Fig. S2 in the Supplemental Materials), respectively.105 After UV irradiation, the fluorescence intensity of Pdots containing 62 and 66 was quenched by 99%, while that of Pdots containing 63 and 66 was only reduced by 45%. The result was ascribed to the highly efficient FRET from polymer 66 to the UV light-triggered open-ring forms of dyes 62 and 63. Based on their excellent photoswitching properties, dual colorimetric and fluorescent authentication were explored. First, the word “Chemistry” was written on white paper by loading three Pdots solutions (Chem: Pdots containing 62 and 64; is: Pdots containing 62 and 65; try: Pdots containing 62 and 66) into the pen. The color and luminescence of the word had reversibly changed upon alternating exposure to UV and visible light owing to the reversible FRET on/off switching from RGB polymers 64 to 66 to dyes 62 [Fig. 4(b)], which indicates that full-color patterns can be successfully created by mixing three Pdots at different proportions. Next, a full-color wheel, personal photograph, and 2D barcode were printed successfully, respectively, by using the mixture of Pdots, glycerol, and ethanol as standard RGB ink. The same color and luminescence changes were achieved [Figs. 4(b)-II, III, and IV], which further suggests that patterns with full spectra can be coordinated and manipulated by mixing the RGB colors. Interestingly, the above patterns were waterproof, and their photoswitching performance remained unchanged after exposure to water, indicating that these photoswitchable Pdots can be applied stably in anticounterfeiting. In order to further explore the practical application of these photoswitchable Pdots in anticounterfeiting, full-color patterns were printed as security marks on personal bank checks so that the checks can be easily verified. This work provides dual colorimetric and fluorescent authentication for customers and retailers and has important guidance for future anticounterfeiting technologies related to document authentication and fraud deterrence. Roghani-Mamaqani and coworkers developed a series of photochromic and photofluorochromic SP-containing latex particles by semicontinuous emulsifier-free emulsion polymerization for anticounterfeiting and photopatterning.70,107,108 The significant particle size variation (aggregation-disaggregation) occurred by UV light irradiation, which resulted from the electrostatic interactions and/or the stacking interactions between the different particles. In addition, the polar interaction between MC molecules and functional groups affected the photoswitching behaviors of these latex particles. Interestingly, these latex particles were directly used as high-security anticounterfeiting inks for writing on security documents and/or general cellulosic papers and/or in photopatterning because the functional groups such as epoxy, hydroxyl, and carboxyl on their surface were attached to the cellulosic paper through physical or chemical interaction, and the large particle size of nanoparticles prevents them from diffusing into the cellulosic fibers. The prepared anticounterfeiting labels and rewritable photopatterns exhibited reversible photochromic and photofluorochromic behaviors as a result of the reversible photoisomerization of SP⇌MC upon alternating irradiation of UV and visible light [Fig. 4(c)]. Anticounterfeiting labels were colorless and nonluminescent under visible light irradiation, while showed red or purple and red emission after UV light irradiation. The rewritable photopatterns on the cellulose paper were created by UV light irradiating different masks, wherein the colored patterns were quickly erased by heat treatment or visible light irradiation. These works offered efficient strategies for preparing high-security polymeric anticounterfeiting inks, which helps to reduce document counterfeiting and copying, and improves their security.
6 Photoresponsive Supramolecules
Molecular self-assembly is widespread in chemical and biological systems.26 The self-assembly process is endowed by the intrinsic dynamic characteristics of noncovalent interactions in supramolecular systems.26 Different types of photochromes can be noncovalently combined into distinct architectures that are assembled based on host–guest interaction, so that functional supramolecular aggregates could be realized without additional synthetic effort.109 Furthermore, highly ordered aggregates can offer enough spatial organization for donors and acceptors to ensure precisely controllable functions and efficient electron and/or energy transfer.109 Its simple construction strategy and effective electron and/or energy transfer platform are very conducive to the design and development of photoresponsive materials for information storage.
6.1 Photoresponsive Small Molecule-Based Self-Assembly
Photoresponsive small molecule-based self-assembly mainly depends on the host–guest-driven self-assembly strategy. Liu and coworkers developed two photocontrollable supramolecular switches as photoerasable fluorescent inks, in which Ru(II) complexes are the hosts and DAE derivatives are the guests.54 One supramolecule was designed by the self-assembly between the Ru(II) complex 67 (Fig. S2 in the Supplemental Materials) appended with two crown ethers moieties and the DAE derivative 68 (Fig. S2 in the Supplemental Materials) containing two dialkylammonium ions. In this system, the crown ethers noncovalently associated with the secondary alkylammonium ion through the hydrogen-bonding interactions of and . Guest 68 underwent the reversible open-closed ring reaction in the solution, solid state, and PMMA film under alternating radiation with 254- and light. The open-ring form (OF-68) was colorless, while the closed-ring form (CF-68) was blue and exhibited strong red emission. In addition, host 67 had a strong emission at . Therefore, the efficient FRET can occur from host 67 to CF-68, resulting in the red luminescence of assembly under light irradiation and the luminescence quenching upon 254-nm light irradiation. Based on its excellent photoswitchable luminescence behavior, the assembly was used as the fluorescence ink for information writing and erasing. Upon 365-nm light irradiation, the information was written on the paper after being dried in air, disappeared upon 254-nm light irradiation, and then completely recovered by irradiating with light. These photocontrolled erasing-recovery cycles were reversible and repeatable. Other supramolecular nanoparticles were fabricated by the noncovalent host–guest and hydrophilic–hydrophobic interactions between -CD-functionalized Ru(II) complex 69 (Fig. S2 in the Supplemental Materials) and DAE derivative 70 (Fig. S2 in the Supplemental Materials).55 These supramolecular nanoparticles were water-soluble and exhibited the same photoswitching behavior as the above system. Therefore, information coding and anticounterfeiting applications were realized using the aqueous solution of supramolecular nanoparticles as ink. Various patterns can be obtained by spraying ink onto filter paper and drying in the air. These patterns were invisible under daylight. With exposure to 365-nm light, the patterns exhibited bright red fluorescence, so that previously hidden information can be read out. The red luminescent patterns completely disappeared after irradiating with 254-nm light for a few minutes and recovered under light irradiation. These supramolecular switches can be excellent candidates for information storage.
6.2 Photoresponsive Polymer-Based Self-Assembly
Photoresponsive polymer-based self-assembly has various construction strategies. Based on the host–guest-driven self-assembly strategy, Wang and coworkers prepared a series of photoresponsive supramolecular nanoparticles as security inks for anticounterfeiting, including water-soluble pillar[5]arene as the host, three conjugated polyfluorene derivatives 71 to 73 (Fig. S2 in the Supplemental Materials) with RGB emission as the guests.109 In addition, benefiting from the noncovalent self-sorting encapsulation, the photochromic DAE derivative 74 (Fig. S2 in the Supplemental Materials) with broad-spectrum tunability as an energy acceptor was spatially positioned at the hydrophobic layer of the formed nanoparticles. The RGB fluorescence of these nanoparticles was exhibited under 365-nm light excitation and was quenched by 254-nm light irradiation because the FRET process occurred from three luminophores 71 to 73 to the closed-ring form of compound 74. Upon 520-nm light irradiation, the RGB fluorescence recovered as photoisomerization switching of compound 74 from the closed-ring form to the open-ring form. Considering the excellent water-soluble and photoswitching characteristics, the anticounterfeiting application was demonstrated by loading the nanoparticles with and without compound 74 as security inks in pens. After irradiating with 254-nm light for 40 s, images containing compound 74 vanished owing to the fluorescence quenching as a result of the photoisomerization of compound 74, and the image without compound 74 was not influenced. The disappearing images were restored by irradiating with visible light for 5 min. Another effective approach for constructing information storage supramolecular materials is to assemble photoresponsive moieties into supramolecular polymers. Gao and coworkers designed and synthesized photoresponsive supramolecular polymers toward fluorescent anticounterfeiting applications, where two monomers 75 and 76 (Fig. S2 in the Supplemental Materials) connected the moiety with an amide unit to the two ends of 9,10-diethyneanthracene and 4,7-benzothiophene, respectively.110 Owing to the σ-metalation effect, anthracene-endoperoxide photoswitching rapidly proceeded under UV light irradiation, and the reverse process occurred spontaneously at room temperature. Due to the cooperative participation of stacking and hydrogen bonding interactions, single- and dual-mode fluorescent anticounterfeiting inks were prepared by the supramolecular polymerization of monomer 75 and the supramolecular coassembly between monomers 75 and 76, respectively. The green luminescence of single-mode anticounterfeiting ink was exhibited under UV light and was quenched upon being irradiated with 460-nm light. Specifically, the monochromic invisible QR code was printed on nonfluorescent paper by inkjet printing and could be decoded by smartphones under UV light. Under 460-nm light irradiation, the QR code was completely erased within 2 min and restored to its original state after being placed at room temperature for 10 min. The dual-mode anticounterfeiting ink benefited from the modulation of FRET from donor 75 to acceptor 76 through alternating light and vapor stimuli. The yellow emission pattern was obtained by inkjet printing on nonfluorescent paper. Upon exposure to 460-nm light, the emission intensity was significantly reduced owing to the deactivation of the FRET effect as a result of photo-oxygenation from anthracene to endoperoxide. The yellow fluorescence then recovered within 4 min at 40°C. These works have enriched highly effective anticounterfeiting materials through the supramolecular polymerization of photochromic molecules.
7 Photoresponsive Cholesteric Liquid Crystals
Photoresponsive CLCs with self-organizing helical superstructures have been widely studied for constructing multicolor patterns and information storage owing to their ability to selectively reflect light. They are usually formed by doping chiral photochromic molecules into achiral nematic liquid crystals (LCs) [Fig. 4(d)].106,111,112 Selective reflection depends on the pitch length () of the helices in the CLCs that are sensitive to light stimulus. Chiral photochromic molecules can twist the nematic LC phase into a helical superstructure, which is named the helical twisting force (HTP, ).82,103,104 The central wavelength () of the selective reflection is calculated by , where is the average refractive index of the CLCs, and HTP is expressed by the formula , where is the chiral photochromic molecules concentration.106,111,112 Therefore, the selective reflection could be regulated through the variations in HTP and the pitch length of the helices, which are caused by the configurational changes of photochromic molecules. Photoresponsive CLCs with high HTP and large HTP changes are the pursuit of scientists. Hitherto, photoresponsive CLCs based on Azo, DAE, and cyanostilbene scaffold are widely studied for information storage.
7.1 Photoresponsive Azobenzene-Based Cholesteric Liquid Crystals
Azo-based chiral switches have attracted great attention due to the huge difference in molecular geometry between bent cis form and rod-like trans form, resulting in large variation in HTP upon photoisomerization. Li and coworkers designed a new chiral Azo derivative 77 (Fig. S3 in the Supplemental Materials) with three configurations (trans, trans), (trans, cis), and (cis, cis) for optically tuning helical superstructures and reversibly dynamically adjusting full range colors.106 Since chiral switch 77 contains two Azo linkages, the reversible cis-trans isomerization induced by UV light irradiation produced two other isomers containing one or two cis configurations, respectively. The HTP value of the CLC containing 6.5 wt. % 76 was at the initial state and sharply decreased at photostationary states (PSS, , ). The reflection color can be reversibly adjusted from the UV region to the NIR region [Fig. 4(e)]. Under the irradiation of 365-nm light, the reflection wavelength was tuned from the UV region to the NIR region within 50 s, while the reverse process was completed in 20 s upon 520-nm light irradiation. Moreover, a light-driven device was successfully fabricated by sandwiching the CLC layer into two transparent electrodes. Information was recorded in a planar state within seconds using a photomask under UV light irradiation, was hidden in focal conic state by applying a 30-V pulse, and reappeared under a 60-V pulse. In addition, two axially chiral azobenzocycloalkanes 78 and 79 (Fig. S3 in the Supplemental Materials) were designed and synthesized as optically tunable chiral dopants to induce helical superstructures in three achiral LC hosts with different structures.111 Since the reversible cis-trans isomerization of cyclic azobenzocycloalkanes under alternating UV and visible light irradiation, these CLCs showed high HTP values and can selectively control the reflection color from blue to NIR. Moreover, the different switching states of 78 and 79 can also result in a helical superstructure of opposite handedness. Subsequently, Li and coworkers also prepared a series of Azo derivatives 80 to 82 (Fig. S3 in the Supplemental Materials) with multiple chiral configurations by introducing axially chiral binaphthyl moieties with opposite chiral configurations.112 Upon 365-nm light irradiation, these molecules underwent cis-trans configuration conversion, which regulated the helical superstructure of various nematic LCs. However, UV light should be avoided in practical applications, as it will cause material decomposition, poor penetration, and strong absorption in the substrate. Li and coworkers realized reversible visible-light tuning of self-organized helical superstructures of the CLCs using axially chiral Azo switches 83-() and 83-() (Fig. S3 in the Supplemental Materials) with extended conjugation.113 They showed HTP values in the initial state, and their HTP values were increased upon visible-light irradiation (, ). Reversible and fast RGB reflections were obtained by mixing 22.7 wt. % 83-() and LC. The RGB reflection color was obtained within about 30 s under 440-nm light irradiation, and the reversible reflection process occurred within 20 s upon 550-nm light irradiation. Yu and coworkers reported axially chiral -fluorinated Azo 84–86 (Fig. S3 in the Supplemental Materials) for visible light regulating the self-organized helical superstructures of CLCs.114 The ortho-fluorine atom caused the separation of the absorption bands of the cis and trans isomers, so that visible light can induce cis-trans or trans-cis isomerization, respectively. These Azo switches all showed high HTP values (100 to ) at the initial state, whereas their HTP values dropped sharply after visible light irradiation ( to , to ). Reversible reflection color was regulated in the full-range visible spectrum by mixing 15.8 wt. % in LC. Upon exposure to 530-nm light for 27 s, the reflection band of CLCs was redshifted to the entire visible light region from the UV region (), and the reflection color was dynamically changed from blue through green to dark red. The reverse process was carried out under 445-nm light irradiation. In addition, green and red patterns were successfully written and erased on the blue background by adjusting the RGB reflection colors of the CLC mixture under alternated irradiation with 530- and 445-nm light. However, full-color reflective display failed to be achieved because the optically addressed image must sacrifice a color in the visible spectrum as the background, which was caused by LCs containing only one kind of photoswitch chiral molecule. Yu and coworkers kept the RGB reflection color of the image in the visible region and further adjusted the reflection in the NIR region to create a black background by combining two different optical switches into a chiral structure.115 Chiral switch 87 (Fig. S3 in the Supplemental Materials) used the binaphthalene moiety as the axial chiral center, and Azo and o-fluoroazobenzene are two photoelectric switches near and far from the chiral center, respectively. Azo underwent reversible cis-trans and trans-cis isomerization upon alternating exposure to UV and visible light, while o-fluoroazobenzene only performed the cis-trans isomerization under UV-visible light irradiation owing to the separation of bands of cis and trans isomers. Therefore, chiral switch 86 possessed three reversible conversion configurations under different wavelengths of light irradiation. Configuration I (trans, trans, trans, trans) and configuration III (cis, cis, cis, cis) were exhibited under the irradiation of 365 and 470 nm, respectively. Upon 530-nm light irradiation, configuration II (cis, trans, trans, cis) was converted from configuration I and configuration III. The right-handed CLC mixture was formed by doping 0.09 mol. % chiral switch 87 into nematic LC host E7. The HTP values of the CLC mixture at the initial state and three PPSs (530, 365, and 470 nm) were 271, 112, 36, and , respectively. Moreover, the HTP values can be manipulated according to the equilibrium ratio of three configurations using composite light. Specifically, HTP values changed in the range of 160 to , and 112 to under the composite light irradiation of 470 and 530 nm and 530 and 365 nm with different intensities, respectively, which were caused by the different ratios of configurations I and II and configurations II and III. The selective reflection of the CLC mixture exhibited two adjacent and continuous tuning periods in the visible and NIR regions. The reflection color was tuned from blue to green then to red under the irradiation of 530-nm light in 23 s, whereas the reverse process was induced upon 470-nm light irradiation in 14 s. The CLC possessed three primary RGB reflection colors in the visible spectrum upon irradiation of 470- and 530-nm light and had a wide tuning range of in the NIR region by irradiation of 530- and 365-nm light. Therefore, colorful patterns with the black background can be created by irradiating the cell with light of different wavelengths through masks. When the reflection color was adjusted to the desired one, patterns were created by irradiating the mask placed on the cell with 365-nm light. The reflection wavelength of the exposed region was further adjusted to the NIR area, prompting the background color to turn into black, whereas the unexposed region still displayed the original color. This work broke the limitation of bistable chiral switches and successfully developed RGB reflection display with a black background. Li and coworkers realized the first reversible NIR light-guided self-organizing helical superstructure by doping Azo derivative 88 (Fig. S3 in the Supplemental Materials) and upconversion nanoparticles (UCNPs) in nematic LCs.116 In this system, reversible cis-trans and trans-cis photoisomerization cycles of 88 were induced only by varying the excitation power density of 980-nm NIR light, which was ascribed to intermolecular energy transfer from the UCNPs to chair switch 88. Specifically, the UV emissions generated from the UCNPs drove the trans-cis isomerization of chair switch 88 at the high power density, while the reverse cis-trans isomerization was triggered by visible light resulting from the same UCNPs at the low power density. The HTP value in the initial state was and decreased to in the PPSs. Reversible dynamic RGB reflections were exhibited in a thin film through modulating the power density of the excitation NIR light. After being irradiated with a high-power density 980-nm NIR laser, the reflection wavelength was changed from 435 nm in the initial state to 540 nm within 60 s and further reached a PSS within 120 s. The reverse process occurred within 4 min at low power density. This work realized the regulation of the self-organized helical superstructure and its dynamic reflection. However, energy transfer between the UCNPs and chair switch 88 resulted in slow response and low photoisomerization efficiency. Wei and coworkers introduced fluorescent dyes with RGB emission colors and chiral Azo derivative 89 (Fig. S3 in the Supplementary Materials) into LCs to construct multicolor patterns with the reflection mode (R-mode) and fluorescent mode (F-mode) [Fig. 5(a)].117 The reflection wavelength of the CLCs was adjusted by varying the content of fluorescent dyes and the photoisomerization degree of chiral switch 89. The luminescence intensity reached its maximum when the emission peak of the fluorescent dyes overlapped with the central reflection wavelength. Dual-mode multicolored patterns containing different 89-CLC/dyes were prepared by the stencil printing method. The patterns showed orange in the R-mode and RGB emission in the F-mode at the initial state. Owing to the photoisomerization of the chiral switch 89, the reflection color became green, and the luminescent intensity decreased after 365-nm light irradiation. Then, the reflection color and luminescent intensity of the patterns returned to the initial state after 450-nm light irradiation or heat treatment. These reversible dual-mode multicolored pattern demonstrations allow CLCs for optical imaging, anticounterfeiting, and color display.
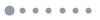
Figure 5.(a) Pattern formation and color change in the R-mode and F-mode. Reproduced with permission from Ref. 117, © 2019 Royal Society of Chemistry. (b) Photographs of dynamic fluorescent anticounterfeiting using LCNPs-based ink. Reproduced with permission from Ref. 118, © 2019 WILEY-VCH Verlag GmbH & Co. KGaA, Weinheim. (c) Real cell images containing 104-() in R-mode (a-e) and F-mode (a’-e’) by irradiating the panda photomask with 365-nm light for different times. Reproduced with permission from Ref. 119, © 2017 WILEY-VCH Verlag GmbH & Co. KGaA, Weinheim. (d) Information encryption and decryption demonstration using CLC microtubes with and without dye 107. Reproduced with permission from Ref. 120, © 2020 WILEY-VCH Verlag GmbH & Co. KGaA, Weinheim.
7.2 Photoresponsive Diarylethene-Based Cholesteric Liquid Crystals
As far as we know, DAE and its derivatives have better fatigue resistance and thermal stability than Azo and its derivatives. However, their application as chiral switches is restricted by low HTP or/and small variations in HTP during photoisomerization.121 Therefore, some excellent works have been reported to improve their HTP value and then realize the application in the field of information storage. Li and coworkers developed DAE-based axially chiral switch 90 (Fig. S3 in the Supplemental Materials) that was connected to two naphthyl groups.121 90-() and 90-() (Fig. S3 in the Supplemental Materials) induced right-handed and left-handed helixes, respectively. The HTP values of 90-() and 90-() in the nematic LC host changed from the initial to by irradiating with 310-nm light. However, upon 550-nm light irradiation, the HTP values were almost changed back to their original state with . Moreover, reversible RGB reflection colors with thermal stability can be implemented by mixing a low concentration (7.7 wt. %) of 90-() in the nematic LC host. The reflection wavelength was about 630 nm in the original state. Under the irradiation of 310-nm light, the reflection wavelength was first blueshifted to 530 nm within 10 s and then further blueshifted to the within 25 s. The was thermally stable and was photochemically converted back to the original state within 2 min by 550-nm light irradiation. A series of dithienylcyclopentene derivatives 91 to 93 containing two axially chiral binaphthyl units with different bridge lengths were designed and synthesized.122 Switches 91 and 92 (Fig. S3 in the Supplemental Materials) exhibited ultrahigh HTPs and a significant reduction in HTP during photoisomerization in different nematic LCs. Meanwhile, switch 93 (Fig. S3 in the Supplemental Materials) in different LC hosts induced a reversible handedness inversion with medium HTPs in initial and PSS states as the bridge length increased. RGB reflection colors were tuned by mixing 2.0 mol. % switch 91 in E7. By UV light irradiation, the reflection wavelength was adjusted from 480 to 550 nm within 20 s and then redshifted to 620 nm. The reverse process was completed in 240 s after 550-nm light irradiation. Moreover, different reflection colors were displayed in the same cell by masking different areas, allowing the information to be written. More similar DAE-based chiral switches 94 to 99 (Fig. S3 in the Supplemental Materials) have been reported for tuning self-organized helical superstructures and reflection colors of CLCs.123,124 In addition, water-dispersible and reversible phototunable fluorescent liquid crystal nanoparticles (LCNPs) were first prepared via a miniemulsion polymerization for information encryption and anticounterfeiting, which consisted of an AIEE-based dicyanodistyrylbenzene monomer 100 as the emitter, a photochromic switch DAE derivative 101 as the quencher, LC monomer 102 as the cross-linking agent, and AC10COONa 103 as the surfactant (Fig. S3 in the Supplemental Materials).118 Nanoparticles showed pale yellow and emitted bright green fluorescence in aqueous suspension. After irradiating with UV light for 30 s, the color changed to deep taupe, and the fluorescence was quenched. The initial state was restored by visible light irradiation for 90 s. Their reversible photoswitchable behavior was achieved by regulating the on and off of FRET from 100 to 101 upon alternating exposure to UV and visible light. The excellent water dispersibility and photoswitchable properties allowed the LCNPs-based security ink for multiple information encryption and anticounterfeiting. Since the ink was colorless under natural light, information was encrypted on the paper in ambient conditions. The hidden information exhibited bright green emission under 365-nm excitation light and showed dark gray-brown upon UV light irradiation, thereby completing information decryption. Therefore, the printed QR code cannot be identified by smartphones under natural light. By observing under UV light, the QR code with bright green fluorescence was presented that can be recognized by a phone. Another strategy for identifying the QR code was to irradiate it with UV light. At this time, the QR code was dark brown and can be easily recognized [Fig. 5(b)]. These LCNPs achieve high-level anticounterfeiting because a sort of QR code can only be identified under some special treatment. Moreover, this work extends the application of photoresponsive LCNPs to many fields including fluorescence sensors, displays, information storage, and other photonic devices.
7.3 Photoresponsive Cyanostilbene Scaffold-Based Cholesteric Liquid Crystals
In addition to Azo and DAE derivatives, chiral switches based on cyanostilbene scaffold have been exploited in the field of transparent displays because they exhibit tunable photoswitching without thermal relaxation under light-driven isomerization. Guo and coworkers first reported two chiral fluorescence switches 104-() and 104-() (Fig. S3 in the Supplemental Materials) based on dicyanodistyrylbenzene enantiomers for constructing optically tunable reflective-photoluminescent CLC devices.119 Two chiral switches in SLC-1717 showed high HTP values at their initial state and exhibited a remarkable decrease in their HTP at , which resulted from the dramatic geometrical change upon UV irradiation. The reflection band of the CLC mixture containing 5.0 wt. % 104-() was tuned from the UV region across the entire visible region to the NIR region within 30 min under the irradiation of 365-nm light. Subsequently, reflective-photoluminescent CLC devices with different reflection and fluorescence colors were successfully fabricated by tuning the irradiation time of 365-nm light. A panda image on the CLC cell was achieved by irradiating a negative photomask with regional light [Fig. 5(c)]. In exposure to 365-nm light for 25 min, panda images with different colors on different backgrounds were presented in the R-mode, and the fluorescence intensity of the panda images on the same backgrounds gradually decreased in the F-mode. The reflection image disappeared, and the panda image with blue fluorescence appeared on the black background after removing the photomask and further irradiating for 5 min. The rich color changes in R- and F-modes were ascribed to the irreversible photoisomerization of 104-(). Based on the above research work, Guo and coworkers further developed two dicyanostilbene-based chiral fluorescent switches 105-() and 106-() (Fig. S3 in the Supplemental Materials) for rewritable transparent display devices.125 Two switches showed reversible photoisomerization and significant changes in fluorescence intensity under alternated 365- and 450-nm light irradiation. Therefore, optically rewritable transparent CLC display devices operating in multiple modes with good transparency, fast response, and high resolution can be realized under all ambient conditions. The display devices operating in the photoluminescent mode were constructed by a low-chiral CLC film containing 1.0 wt. % 105-() in SLC-1717 media. A transparent CLC screen was created by irradiating the whole cell with 450-nm LED light to reach PSS450. Subsequently, the desired information was written by a UV-light-emitting digital projector or using either 365-nm UV light passing through patterned photomasks. Fluorescent information was successfully written in the CLC device by 365-nm light irradiation, was erased by 450-nm LED light, and then rewritten under 365-nm light irradiation. The writing-erasing process can be repeated many times. Furthermore, the CLC display device operating in the R-mode or F-mode was fabricated by increasing the concentration of 105-() to 13.8 wt. % in SLC-1717 media for simultaneous tuning of the fluorescent intensity and reflection wavelength upon exposure to 365- and 450-nm light. The CLC device was colorless and highly transparent in two modes at the PSS450 state. The information was written at the PSS450 state after irradiating the photomask with 365-nm light. Information with yellow-green fluorescence was displayed under a 450-nm light source, whereas that with an orange reflection color was clearly observed under white light. Therefore, the rewritable CLC display devices were switched between the F-mode in darkness and the R-mode under bright condition on-demand operation. In addition, the written information was partially or completely erased by 450-nm light irradiation, and was easily modified multiple times. Interestingly, information can be also remotely written to the CLC display device by a handheld 365-nm laser for achieving a “remote-writing board.” This work provides a promising way to construct multimode optical rewritable display devices with instantaneous remote writing capability. Furthermore, Guo and coworkers introduced dynamic FRET into the nematic LC host for the first time to regulate the fluorescent color, in which a conventional coumarin dye 107 (Fig. S3 in the Supplemental Materials) was a donor, and a chiral fluorescent photoswitch 108 (Fig. S3 in the Supplemental Materials) based on 1,2-dithienyldicyanoethene was an acceptor.120 The FRET of the CLC mixture was modulated by the reversible cis-trans photoisomerization of switch 108 under alternating irradiation of UV and visible light. Initially, self-organized helical superstructures with orange-red emission were formed, accompanied by the occurrence of FRET. However, under 520-nm light irradiation, self-organized helical superstructures showed green emission because the FRET process was inhibited. Finally, self-organized helical superstructures exhibited orange emission again since the FRET process was reactivated by 365-nm light irradiation. Phototunable CLC cells were fabricated for information display. High contrast fluorescent information was written on the cell using photomask technology and was erased by light irradiation at 520 or 365 nm. Information encryption and decryption are accomplished using CLC samples with and without dye 107 in the microtubes [Fig. 5(d)]. The initial number “88888” array made from two kinds of microtubes was easily converted into letter “HELLO” after being irradiated by 520-nm light, in which the emission color of CLC microtubes containing dyes 107 and 108 changed from orange-red to yellow-green, and the emissive intensity of CLC microtubes doped only by switch 108 decreased. This work provides a new avenue for the development of photoswitchable fluorescent CLC materials for information storage.
8 Conclusion and Outlook
During the past decades, significant progress has been made in the development of organic photoresponsive materials for information storage. Organic photoresponsive materials based on various kinds of photochromes are designed and synthesized and have been successfully applied to various fields of information storage, such as information writing and erasure, encryption and decryption, and anticounterfeiting. These successful applications not only increase the safety of daily life and commercial activities but also greatly reduce environmental pollution. In this review, we systematically summarized the design strategies and photoswitching mechanisms of various photoresponsive materials for information storage, including small organic molecules, metal complexes, polymers, supramolecules, and CLCs. Subsequently, we also introduced in detail their applications in information (re)writing and erasing, encryption and decryption, and anticounterfeiting. The objectives of this review are to present the current research achievement and future development on photoresponsive materials for information storage. Compared with other organic optoelectronic fields, although significant progress has been achieved on this field, information storage applied by photoresponsive materials is still at an early stage, and there are some problems and challenges. For the existing photoresponsive materials, there are also problems for which the photoswitching mechanisms are too simple, and the molecular species and structures are not rich enough. Most materials only involve the conversion of two configurations under different wavelengths of light irradiation, making the realization of advanced information storage difficult. Compared with photochromic counterparts, organic materials that simultaneously exhibit photochromism and fluorescent photoswitching can significantly improve the level of information storage. However, most of them only achieve the single-color fluorescence on/off photoswitching. Multistate photoswitching with both color and fluorescence changes is the key to achieving advanced and multilevel information storage. Therefore, the design and development of organic photoresponsive materials for information storage should follow the following future directions.
In spite of the huge challenges faced by organic photoresponsive materials for information storage, we believe that the advanced and multilevel information storage will be realized as soon as possible and will bring much convenience to human life via resolving the above-mentioned scientific and technical problems.
References
[1] Y. Zhuang et al. Optical data storage and multicolor emission readout on flexible films using deep-trap persistent luminescence materials. Adv. Funct. Mater., 28, 1705769(2018).
[2] M. Gu et al. Optical storage arrays: a perspective for future big data storage. Light: Sci. Appl., 3, e177(2014).
[3] M. Gu et al. Nanomaterials for optical data storage. Nat. Rev. Mater., 1, 16070(2016).
[4] D. Liu et al. Tailoring multidimensional traps for rewritable multilevel optical data storage. ACS Appl. Mater. Interfaces, 11, 35023-35029(2019).
[5] X. Ouyang et al. Polychromatic and polarized multilevel optical data storage. Nanoscale, 11, 2447-2452(2019).
[6] D. Xu et al. Multi-Dimensional Optical Storage(2016).
[7] L. Wang et al. Photochromism into nanosystems: towards lighting up the future nanoworld. Chem. Soc. Rev., 47, 1044-1097(2018).
[8] J. Zhang et al. Photochromic materials: more than meets the eye. Adv. Mater., 25, 378-399(2012).
[9] D. Kim et al. Multicolor fluorescence photoswitching: color-correlated versus color-specific switching. Adv. Opt. Mater., 6, 1800678(2018).
[10] D.-H. Qu et al. Photoresponsive host-guest functional systems. Chem. Rev., 115, 7543-7588(2015).
[11] Y. Wu et al. Crystal structure and phototransistor behavior of N-substituted heptacence. ACS Appl. Mater. Interfaces, 4, 1883-1886(2012).
[12] G. Sonmez et al. A highly stable, new electrochromic polymer: poly(1,4-bis(2-(3′,4′-ethylenedioxy)thienyl)-2-methoxy-5-2″-ethylhexyloxybenzene). Adv. Funct. Mater., 13, 726-731(2003).
[13] Z. Li et al. Loading photochromic molecule into luminescent metal-organic framework for potential information anti-counterfeiting. Angew. Chem. Int. Ed., 58, 18025-18031(2019).
[14] Y. Li et al. Recent advances in organic-based materials for resistive memory applications. Infomat, 2, 995-1033(2020).
[15] B. Zhang et al. Redox gated polymer memristive processing memory unit. Nat. Commun., 10, 736(2019).
[16] T. Leydecker et al. Flexible non-volatile optical memory thin-film transistor device with over 256 distinct levels based on an organic bicomponent blend. Nat. Nanotechnol., 11, 769-775(2016).
[17] P.-Y. Gu et al. Synthesis of tetranitro-oxacalix[4]arene with oligoheteroacene groups and its nonvolatile ternary memory performance. Mater. Horiz., 1, 446-451(2014).
[18] B. Hwang et al. Recent advances in memory devices with hybrid materials. Adv. Electron. Mater., 5, 1800519(2019).
[19] C. Wang et al. Recent progress on organic resistance memory with small molecules and inorganic-organic hybrid polymers as active elements. J. Mater. Chem. C., 3, 10055-10065(2015).
[20] S. Goswami et al. Nanometer-scale uniform conductance switching in molecular memristors. Adv. Mater., 32, 2004370(2020).
[21] M.-M. Russew et al. Photoswitches: from molecules to materials. Adv. Mater., 22, 3348-3360(2010).
[22] H. Bouas-Laurent et al. Organic photochromism (IUPAC technical report). Pure Appl. Chem., 73, 639-665(2001).
[23] M. Irie et al. Photochromism of diarylethene molecules and crystals: memories, switches, and actuators. Chem. Rev., 114, 12174-12277(2014).
[24] R. Pardo et al. Photochromic organic-inorganic hybrid materials. Chem. Soc. Rev., 40, 672-687(2011).
[25] D. Bléger et al. Visible-light-activated molecular switches. Angew. Chem. Int. Ed., 54, 11338-11349(2015).
[26] X. Yao et al. Recent progress in photoswitchable supramolecular self-assembling systems. Adv. Opt. Mater., 4, 1322-1349(2016).
[27] J. Zhang et al. The endeavor of diarylethenes: new structures, high performance, and bright future. Adv. Opt. Mater., 6, 1701278(2018).
[28] Q. Yan et al. The marriage between aggregation induced emission and photochromics for promising photoresponsive smart materials. Mater. Chem. Front., 4, 3153-3175(2020).
[29] Q. Zhang et al. Nondestructive up-conversion readout in Er/Yb co-doped Na0.5Bi2.5Nb2[30] K. Li et al. Polymer encapsulated conjugated polymer nanoparticles for fluorescence bioimaging. J. Mater. Chem., 22, 1257-1264(2012).
[31] J. Chen et al. Design and synthesis of FRET-mediated multicolor and photoswitchable fluorescent polymer nanoparticles with tunable emission properties. J. Phys. Chem. B., 116, 4354-4362(2012).
[32] H. Wang et al. Switchable single fluorescent polymeric nanoparticles for stable white-light generation. J. Mater. Chem. C, 6, 9897-9902(2018).
[33] H. Gao et al. Site isolation of emitters within cross-linked polymer nanoparticles for white electroluminescence. Nano Lett., 10, 1440-1444(2010).
[34] Z. Tian et al. Photoswitching-enabled novel optical imaging: innovative solutions for real-world challenges in fluorescence detections. Acc. Chem. Res., 46, 269-279(2013).
[35] Z. Xu et al. Supramolecular color-tunable photoluminescent materials based on a chromophore cascade as security inks with dual encryption. Mater. Chem. Front., 1, 1847-1852(2017).
[36] J. Cusido et al. Fluorescent switches based on photochromic compounds. Eur. J. Org. Chem., 2009, 2031-2045(2009).
[37] F. M. Raymo et al. Electron and energy transfer modulation with photochromic switches. Chem. Soc. Rev., 34, 327-336(2005).
[38] R. Haldar et al. Advanced photoresponsive materials using the metal-organic framework approach. Adv. Mater., 32, 1905227(2020).
[39] A. M. Rice et al. Photophysics modulation in photoswitchable metal-organic frameworks. Chem. Rev., 120, 8790-8813(2020).
[40] F. Ercole et al. Photo-responsive systems and biomaterials: photochromic polymers, light-triggered self-assembly, surface modification, fluorescence modulation and beyond. Polym. Chem., 1, 37-54(2010).
[41] M. Irie et al. Photochromism of diarylethene single molecules and single crystals. Photochem. Photobiol. Sci., 9, 1535-1542(2010).
[42] Q. Luo et al. Recent progress on photochromic diarylethene polymers. Polym. Chem., 2, 2435-2443(2011).
[43] D. Gust et al. Data and signal processing using photochromic molecules. Chem. Commun., 48, 1947-1957(2012).
[44] M. Qin et al. Photochromic sensors: a versatile approach for recognition and discrimination. J. Mater. Chem. C, 3, 9265-9275(2015).
[45] A. Fihey et al. Multiphotochromic molecular systems. Chem. Soc. Rev., 44, 3719-3759(2015).
[46] E. Orgiu et al. 25th anniversary article: organic electronics marries photochromism: generation of multifunctional interfaces, materials, and devices. Adv. Mater., 26, 1827-1845(2014).
[47] R. Klajn et al. Spiropyran-based dynamic materials. Chem. Soc. Rev., 43, 148-184(2014).
[48] M. Irie et al. Thermally irreversible photochromic systems. Reversible photocyclization of diarylethene derivatives. J. Org. Chem., 53, 803-808(1988).
[49] F. Yu et al. Photostimulus-responsive large-area two-dimensional covalent organic framework films. Angew. Chem. Int. Ed., 58, 16101-16104(2019).
[50] D. Kim et al. Flexible molecular-scale electronic devices composed of diarylethene photoswitching molecules. Adv. Mater., 26, 3968-3973(2014).
[51] A. M. Asadirad et al. Controlling a polymer adhesive using light and a molecular switch. J. Am. Chem. Soc., 136, 3024-3027(2014).
[52] B. Yoon et al. Recent functional material based approaches to prevent and detect counterfeiting. J. Mater. Chem. C, 1, 2388-2403(2013).
[53] M. Natalia et al. Molecular switches as photocontrollable “smart” receptors. Chem. Soc. Rev., 41, 4010-4029(2012).
[54] H. Wu et al. Reversibly photoswitchable supramolecular assembly and its application as a photoerasable fluorescent ink. Adv. Mater., 29, 1605271(2017).
[55] H.-G. Fu et al. Quaternary supramolecular nanoparticles as a photoerasable luminescent ink and photocontrolled cell-imaging agent. Adv. Opt. Mater., 8, 2000220(2020).
[56] G. Hartley et al. The cis-form of azobenzene. Nature., 140, 281(1937).
[57] M. Dong et al. Red-shifting azobenzene photoswitches for in vivo use. Acc. Chem. Res., 48, 2662-2670(2015).
[58] K. E. Sapsford et al. Materials for fluorescence resonance energy transfer analysis: beyond traditional donor-acceptor combinations. Angew. Chem. Int. Ed., 45, 4562-4589(2006).
[59] L. Giordano et al. Diheteroarylethenes as thermally stable photoswitchable acceptors in photochromic fluorescence resonance energy transfer (pcFRET). J. Am. Chem. Soc., 124, 7481-7489(2002).
[60] M. Yu et al. From a molecular toolbox to a toolbox for photoswitchable fluorescent polymeric nanoparticles. Adv. Funct. Mater., 28, 1804759(2018).
[61] J. Han et al. Recent progress on circularly polarized luminescent materials for organic optoelectronic devices. Adv. Opt. Mater., 6, 1800538(2018).
[62] W. Jeong et al. Full color light responsive diarylethene inks for reusable paper. Adv. Funct. Mater., 26, 5230-5238(2016).
[63] G. Bretel et al. Fabrication of robust spatially resolved photochromic patterns on cellulose papers by covalent printing for anticounterfeiting applications. ACS Appl. Polym. Mater., 1, 1240-1250(2019).
[64] N.-H. Xie et al. Deciphering erasing/writing/reading of near-infrared fluorophore for nonvolatile optical memory. ACS Appl. Mater. Interfaces, 11, 23750-23756(2019).
[65] D. Kim et al. Fully reversible multistate fluorescence switching: organogel system consisting of luminescent cyanostilbene and turn-on diarylethene. Adv. Funct. Mater., 28, 1706213(2018).
[66] C. Li et al. A trident dithienylethene-perylenemonoimide dyad with super fluorescence switching speed and ratio. Nat. Commun., 5, 5709(2014).
[67] G. Liu et al. Controlled photoerasable fluorescent behaviors with dithienylethene-based molecular turnstile. ACS Appl. Mater. Interfaces, 10, 12135-12140(2018).
[68] L. Ma et al. Unsymmetrical photochromic bithienylethene-bridge tetraphenylethene molecular switches: synthesis, aggregation-induced emission and information storage. Chin. Chem. Lett., 31, 361-364(2020).
[69] C. Li et al. Photoswitchable aggregation-induced emission of a dithienylethene-tetraphenylethene conjugate for optical memory and super-resolution imaging. RSC Adv., 3, 8967-8972(2013).
[70] A. Abdollahi et al. Rewritable anticounterfeiting polymer inks based on functionalized stimuli-responsive latex particles containing spiropyran photoswitches: reversible photopatterning and security marking. ACS Appl. Mater. Interfaces, 10, 39279-39292(2018).
[71] Q. Qi et al. Solid-state photoinduced luminescence switch for advanced anticounterfeiting and super-resolution imaging applications. J. Am. Chem. Soc., 139, 16036-16039(2017).
[72] S. Sumiya et al. Spiropyran-cholesterol conjugate as a photoresponsive organogelator. New J. Chem., 37, 2642-2647(2013).
[73] Y. Hong et al. Aggregation-induced emission. Chem. Soc. Rev., 40, 5361-5388(2011).
[74] R. Hu et al. Recent advances in AIE polymers. Polym. J., 48, 359-370(2016).
[75] D. D. La et al. Tetraphenylethylene-based AIE-active probes for sensing applications. ACS Appl. Mater. Interfaces, 10, 12189-12216(2018).
[76] Z. Yang et al. Recent advances in mechano-responsive luminescence of tetraphenylethylene derivatives with aggregation-induced emission properties. Mater. Chem. Front., 2, 861-890(2018).
[77] S. Peng et al. Synthesis and application of reversible fluorescent photochromic molecules based on tetraphenylethylene and photochromic groups. New J. Chem., 43, 617-621(2019).
[78] C. Y. Y. Yu et al. A tetraphenylethene-based caged compound: synthesis, properties and applications. Chem. Commun., 50, 8134-8136(2014).
[79] G. Huang et al. Multiple anti-counterfeiting guarantees from a simple tetraphenylethylene derivative—high-contrasted and multi-state mechanochromism and photochromism. Angew. Chem. Int. Ed., 58, 17814-17819(2019).
[80] Y. Ma et al. On-demand regulation of photochromic behavior through various counterions for high-level security printing. Sci. Adv., 6, eaaz2386(2020).
[81] Z. Li et al. Photoresponsive luminescent polymeric hydrogels for reversible information encryption and decryption. Adv. Sci., 6, 1901529(2019).
[82] C. Liu et al. Tunable structural color patterns based on the visible-light-responsive dynamic diselenide metathesis. Adv. Mater., 32, 1907569(2020).
[83] W. Wang et al. Photocatalytic colour switching of redox dyes for ink-free light-printable rewritable paper. Nat. Commun., 5, 5459(2014).
[84] R. Samanta et al. Mechanical actuation and patterning of rewritable crystalline monomer-polymer heterostructures via topochemical polymerization in a dual-responsive photochromic organic material. ACS Appl. Mater. Interfaces, 12, 16856-16863(2020).
[85] J. Yuan et al. Dynamic anti-counterfeiting security features using multicolor dianthryl sulfoxides. Chem. Sci., 10, 10113-10121(2019).
[86] Y. Zhang et al. Excitation wavelength-dependent fluorescence of an ESIPT triazole derivative for amine sensing and anti-counterfeiting applications. Angew. Chem. Int. Ed., 58, 8773-8778(2019).
[87] K. Li et al. Reversible photochromic system based on rhodamine B salicylaldehyde hydrazone metal complex. J. Am. Chem. Soc., 136, 1643-1649(2014).
[88] Y. Li et al. A photoactivatable photochromic system served as self-hidden information storage material. Mater. Chem. Front., 1, 2356-2359(2017).
[89] Y. Li et al. Fluoran salicylaldehyde hydrazone Zn(II) complex: reversible photochromic system both in solution and in solid matrix. J. Mater. Chem. C, 5, 7553-7560(2017).
[90] R. A. Kopelman et al. Photoprocesses and magnetic behavior of photochromic transition metal indoline[phenanthrolinospirooxazine] complexes: tunable photochromic materials. Inorg. Chim. Acta, 361, 3570-3576(2008).
[91] R. A. Kopelman et al. Tunable photochromism of spirooxazines via metal coordination. J. Am. Chem. Soc., 125, 13684-13685(2003).
[92] W.-P. Lin et al. Polymer-based resistive memory materials and devices. Adv. Mater., 26, 570-606(2014).
[93] J.-M. Schumers et al. Light-responsive block copolymers. Macromol. Rapid Commun., 31, 1588-1607(2010).
[94] Y. Zhao et al. Light-responsive block copolymer micelles. Macromolecules, 45, 3647-3657(2012).
[95] P. Theato et al. Stimuli responsive materials. Chem. Soc. Rev., 42, 7055-7056(2013).
[96] J. Wang et al. Organoboron-based photochromic copolymers for erasable writing and patterning. Macromolecules, 50, 4629-4638(2017).
[97] B. Wang et al. High strength dual-crosslinked hydrogels with photo-switchable color changing behavior. Eur. Polym. J., 116, 545-553(2019).
[98] X. Zhang et al. Multi-responsive hydrogel actuator with photo-switchable color changing behaviors. Dyes Pigm., 174, 108042(2020).
[99] P. Chen et al. White-light-emitting lanthanide metallogels with tunable luminescence and reversible stimuli-responsive properties. J. Am. Chem. Soc., 137, 11590-11593(2015).
[100] L. D. Carlos et al. Progress on lanthanide-based organic-inorganic hybrid phosphors. Chem. Soc. Rev., 40, 536-549(2011).
[101] J.-C. G. Bünzli et al. Lanthanide luminescence for biomedical analyses and imaging. Chem. Rev., 110, 2729-2755(2010).
[102] L. Du et al. From shape and color memory PCL network to access high security anti-counterfeit material. Polymer, 172, 52-57(2019).
[103] Z. Lin et al. Photoswitchable ultrahigh-brightness red fluorescent polymeric nanoparticles for information encryption, anti-counterfeiting and bioimaging. J. Mater. Chem. C, 7, 11515-11521(2019).
[104] J. Chen et al. Amphiphilic BODIPY-based photoswitchable fluorescent polymeric nanoparticles for rewritable patterning and dual-color cell imaging. Macromolecules, 48, 3500-3508(2015).
[105] W.-K. Tsai et al. Dual colorimetric and fluorescent authentication based on semiconducting polymer dots for anticounterfeiting applications. ACS Appl. Mater. Interfaces, 9, 30918-30924(2017).
[106] J. Ma et al. Light-driven nanoscale chiral molecular switch: reversible dynamic full range color phototuning. Chem. Commun., 46, 3463-3465(2010).
[107] A. Abdollahi et al. Photoswitchable fluorescent polymer nanoparticles as high-security anticounterfeiting materials for authentication and optical patterning. J. Mater. Chem. C, 8, 5476-5493(2020).
[108] A. Abdollahi et al. Interaction of photoswitchable nanoparticles with cellulosic materials for anticounterfeiting and authentication security documents. Carbohyd. Polym., 230, 115603(2020).
[109] M. Zuo et al. Full-color tunable fluorescent and chemiluminescent supramolecular nanoparticles for anti-counterfeiting inks. ACS Appl. Mater. Interfaces, 10, 39214-39221(2018).
[110] Z. Gao et al. Cooperative supramolecular polymers with anthracene-endoperoxide photo-switching for fluorescent anti-counterfeiting. Nat. Commun., 9, 3977(2018).
[111] M. Mathews et al. Light-driven reversible handedness inversion in self-organized helical superstructures. J. Am. Chem. Soc., 132, 18361-18366(2010).
[112] Y. Li et al. Azoarenes with opposite chiral configurations: light-driven reversible handedness inversion in self-organized helical superstructures. Angew. Chem. Int. Ed., 52, 8925-8929(2013).
[113] Y. Wang et al. Reversible visible-light tuning of self-organized helical superstructures enabled by unprecedented light-driven axially chiral molecular switches. J. Am. Chem. Soc., 134, 3342-3345(2012).
[114] L. Qin et al. Efficient visible-light full-color tuning of self-organized helical superstructures enabled by fluorinated chiral switches. RSC Adv., 8, 38935-38940(2018).
[115] L. Qin et al. Piecewise phototuning of self-organized helical superstructures. Adv. Mater., 30, 1704941(2017).
[116] L. Wang et al. Reversible near-infrared light directed reflection in a self-organized helical superstructure loaded with upconversion nanoparticles. J. Am. Chem. Soc., 136, 4480-4483(2014).
[117] L. Xu et al. Fabrication of multicolored patterns based on dye-doped cholesteric liquid crystals. Photochem. Photobiol. Sci., 18, 1638-1648(2019).
[118] J. Li et al. Photoswitchable fluorescent liquid crystal nanoparticles and their inkjet-printed patterns for information encrypting and anti-counterfeiting. Part. Part. Syst. Charact., 36, 1900346(2019).
[119] J. Li et al. Dicyanodistyrylbenzene-based chiral fluorescence photoswitches: an emerging class of multifunctional switches for dual-mode phototunable liquid crystals. Adv. Opt. Mater., 5, 1700014(2017).
[120] S. Li et al. Phototuning energy transfer in self-organized luminescent helical superstructures for photonic applications. Adv. Opt. Mater., 8, 2000107(2020).
[121] Y. Li et al. Reversible light-directed red, green, and blue reflection with thermal stability enabled by a self-organized helical superstructure. J. Am. Chem. Soc., 134, 9573-9576(2012).
[122] Y. Li et al. Photodynamic chiral molecular switches with thermal stability: from reflection wavelength tuning to handedness inversion of self-organized helical superstructures. Angew. Chem. Int. Ed., 52, 13703-13707(2013).
[123] Y. Li et al. Photochemically reversible and thermally stable axially chiral diarylethene switches. Org. Lett., 14, 4362-4365(2012).
[124] Y. Li et al. A photoswitchable and thermally stable axially chiral dithienylperfluorocyclopentene dopant with high helical twisting power. J. Mater. Chem. C, 1, 3917-3923(2013).
[125] J. Li et al. Optically rewritable transparent liquid crystal displays enabled by light-driven chiral fluorescent molecular switches. Adv. Mater., 31, 1807751(2019).