
- Journal of Inorganic Materials
- Vol. 36, Issue 9, 1006 (2021)
Abstract
Bioactive glasses (BGs) are considered as 3rd generation materials, which exhibit excellent biocompatibility, bioactivity, and osteoinductive activity. BG’s degradation products can promote growth factor production, cell proliferation, osteogenic and angiogenic gene expression, and thus enhance bone tissue regeneration[
It has been widely accepted that the interaction of BG surfaces with body fluids starts with an ion exchange of alkali and alkaline-earth ions with protons from body fluid, which leads to an increase of pH of the medium, followed by the formation of a silica-rich layer and then by the growth of a calcium-phosphate rich layer on the BG surface[
To demonstrate the hypothesis, the in vitro degradation, mineralization, and ion release behavior of the melt-derived borosilicate bioactive glass particles were investigated under an external DC electric field with a lower current. As a comparatively new bioactive glass class, borate-based bioactive glasses have received considerable interest due to their controlled and complete degradation behavior[
1 Materials and methods
1.1 Materials
Boric acid (H3BO3), calcium carbonate (CaCO3), silicon dioxide (SiO2), sodium carbonate anhydrous (Na2CO3), potassium carbonate (K2CO3), magnesoum carbonate hydroxide ((MgCO3)4·Mg(OH)2·5H2O), ammonium dihydrogen phosphate (NH4H2PO4) were purchased from Sinopharm Chemical Reagent Co., Ltd (Shanghai, China). All chemical reagents were chemical grade.
1.2 Preparation of borosilicate glass particles
A borosilicate bioactive glass composition was selected as 18SiO2-6Na2O-8K2O-8MgO-22CaO- 2P2O5-36B2O3[
Glass samples were prepared by melting reagent grade chemicals in a Pt crucible at 1150 ℃ for 1 h, and the melt was poured onto a cold stainless steel plate. The obtained glass was crushed and sieved to give particles sizes in the range of 0.15-3.00 mm.
1.3 In vitro mineralization of borosilicate bioactive glass
Simulated body fluid (SBF) with ion concentration close to that of human plasma was configured according to the method described by Kokubo[
Figure 1.Scheme of DC electric field
The conversion of borosilicate glasses to hydroxyapatite (HA) in the SBF is accompanied by a decrease in the mass of the glass. After each immersion, the samples were removed from SBF, washed with deionized water, and dried at 90 ℃. The weight loss (α) was calculated according to Eq. (1),
where W is the mass of the collected sample and W0 is the initial mass of the glass particles. The weight loss was measured after removing the sample from the SBF, washing, and drying with deionized water in order to keep the precision.
The concentrations of the ions released from the borosilicate bioactive glass particles during degradation were analyzed by inductively coupled plasma-atomic emission spectroscopy (ICP-AES, Optima 2100DV, USA). The structure of glass was determined by Fourier Transform Infrared Spectrometer (FTIR, EQUINOX 55) and X-ray photoelectron spectroscope (XPS, Thermo ESCALAB 250XI). The formation of calcium-phosphate rich layer on glass surfaces was determined by X-ray diffraction (XRD, Smartlab9, Rigaku Co., Japan) and a field emission scanning electron microscope (FESEM, Quanta200FEG).
2 Results and discussion
2.1 Effect of DC field on the degradation rate of borosilicate glass
During the immersion in SBF, the borosilicate bioactive glass particles were attacked by the surrounding environment, and the network-modifying ions were leached out from the glass, which led to structural loosening. B and Si ions, the network formers, were continuously leached out as well. As a result, the glass particles gradually degraded, accompanied by weight loss. The weight loss of the glass particles increased apparently with time (Fig. 2(a)), indicating that the borosilicate glass continuously degraded in SBF during 3 d of immersion. Furthermore, the application of DC field promoted the degradation of the glass particles. The total weight loss increased by 3%-5% compared to the case without the electric field. The weight loss of the glass particles reached a maximum when a current of 60 mA was applied, and further increase of the applied current to 90 mA led to a slight decrease of the weight loss. The increased release of Ca2+ caused faster precipitation of the reactive layer, in turn hindering further release of ions from the glass particles.
Figure 2.Weight loss (a) and comparison of cumulative ion concentration (b) of borosilicate bioactive glass immersed in SBF with different currents applied for 3 d at 37 ℃
The ions released from the glass particles were quantified by ICP-OES (Fig. 2(b)). As expected, the dissolution rate of B and Ca ions is substantially enhanced when various magnitudes of current flew through the SBF, comparing to the control group. Besides, the current of 60 mA exhibited the most pronounced enhancement effect, and in this case the cumulative amount of B and Ca ions released increased ~3 and 2.3 times, respectively. While released Si ions was relatively low, its cumulative released amount still increased by two times. It appears to be evident that the external electric field acts as an additional driving force to assist the concentration gradient-driven ion exchange of alkali and alkaline-earth ions from the glass with the proton ions from the SBF, and thus accelerates the degradation and ion release of the borosilicate bioactive glass.
2.2 Effect of DC field on in vitro mineralization of the borosilicate glass particles
The effect of electric field on the in vitro mineralization behavior of the borosilicate bioactive glass was assessed by immersion in SBF and evaluation of the HA forming ability. The phase composition of the mineralized products was analyzed by XRD after 14 d of immersion in SBF. As shown in Fig. 3, the sample without application of electric field presents a typical amorphous diffraction pattern, presumably because only small amount of reaction products was formed. Upon application of electric field for 1 h per day, the diffraction peaks corresponding to standard HA Ca10(PO4)6(OH)2 (JCPDS 72-1243) were clearly detected, suggesting electric field apparently promoted the conversion of glass to HA. The HA diffraction peak intensity rose with applied current increasing from 30 to 90 mA, indicating a more pronounced enhancement effect. In vitro formation of HA on the glass surface is usually taken as an indicator for in vivo bioactivity. Accordingly, the application of external electric field has a positive effect on the bioactivity of the bioactive glass. Moreover, it is noticeable that under the current of 90 mA, a small amount of CaCO3 crystals (JCPDS 99-0022) was also formed during the mineralization process. This formation is the result of the reaction between Ca2+ released from the glass and CO2 dissolved into SBF.
Figure 3.XRD patterns of bioactive glass immersed in SBF with an applied electric field for 14 d
Fig. 4 shows the surface morphologies of the glass particles after immersion in SBF for 3 d. As shown in Fig. 4(a1), abounding cracks were formed on the surfaces of the glass particles, indicating that the glass surface has been progressively corroded. A higher magnification SEM image (Fig. 4(a2)) shows the surface of the sample is covered by agglomerated tiny particles. The corresponding EDS spectrum (Fig. 4(a3)) confirmed that the Ca/P of the precipitations was 1.13, suggesting the formation of calcium deficient apatite precipitates. Upon exposure to electric fields, more severe corrosion occurred. Comparison of Fig. 4(b2-d2) revealed that larger applied current resulted in more compact HA deposition, and the crystals changed from granular, spherical to bone-like needle-shaped morphology. The relatively dense apatite surface layer acts as a diffusion barrier leading to decreased ion release, as evidenced in Fig. 2(b). Meanwhile, with the growth of applied currents from 30 to 90 mA, the Ca/P of the mineralized products generally increased to 1.66, which is quite close to that of stoichiometric HA. This evidences that crystallization process is enhanced due to the influence of electric field stimulation. The results above highlight that the application of DC field promote the degradation and mineralization of the borosilicate bioactive glass.
Figure 4.Microscopic morphologies of borosilicate bioactive glass immersed in SBF for 3 d after applying electric field and EDS analysis of the formed HA(a1, a2, a3) Without electric field; (b1, b2, b3) 30 mA; (c1, c2, c3) 60 mA; (d1, d2, d3) 90 mA
In the body fluid and SBF environments, Si-O-M and B-O-M (where M is modifying ion) in borosilicate glass undergo bond breaking, alkali and alkaline-earth ions are rapidly dissolved. The negatively-charged surface can link to hydrogen ions in the SBF, making the surface hydroxylation. Subsequently, the deprotonation of the OH group leads to a negatively-charged glass surface, which attracts Ca2+ dissolved from the glass. Ca2+ combines with PO43- in the SBF to form a HA layer afterwards[
Samples were analyzed by FT-IR spectra after 1 d of immersion in SBF to study the structural change of borosilicate glass at the early stage of mineralization. During this period of immersion, several magnitudes of electric current were applied continuously for 15 h. As shown in Fig. 5, for the original glass, the IR band around 715 cm-1 is assigned to bending vibration of B-O-B linkages, and the broad band around 1400 cm-1 corresponds to asymmetric stretching vibration of B-O bonds of trigonal [BO3] units, respectively. The broad band at wavenumbers range of 800-1200 cm-1 is attributed to a combination of vibration modes from Si-O-Si asymmetric stretching, B-O stretching vibration in BO4 units, B-O-M (where M is modifying ions) as well as B-O-Si and B-O-B linkages[
Figure 5.FT-IR spectra of bioactive glass immersed in SBF with an applied electric field
Meanwhile, Fig. 5 showed that the broad band in the 800-1200 cm-1 range sharpened and shifted to higher wavenumber. Especially, a shoulder at 920 cm-1, corresponding to Si-O and B-O vibration, disappeared after the immersion in SBF. The change is indicative of metal cations connected to non-bridging oxygen atoms (nbO) being released (ion exchange) and OH groups forming[
To further clarify the variation of the chemical composition at initial immersion stage, the surface of the glass particles immersed in SBF for 1 d was analyzed by XPS, and results expressed in atomic percentage are shown in Table 1 and Fig. 6. Because of the low sensitivity of XPS to K, only Ca, P, Mg, Na, Si, and B were analyzed compositionally. Ca and P atomic percentage on the glass surface appear to be significantly higher than the calculated values from original glass, especially for the sample exposed to electric field. Such a high Ca/P ratio illustrates the formation of Ca-enriched phosphate precipitation. This is probably because the negatively-charged glass surface by deprotonation of the OH groups, attracted Ca2+ and Mg2+ in the SBF. The cations on the surface will further attract HPO42- and PO43- in SBF to form apatite[
Ca | P | Mg | Na | Si | B | |
---|---|---|---|---|---|---|
0 mA | 16.55 | 8.79 | 7.98 | 5.36 | 26.65 | 34.67 |
60 mA | 21.10 | 10.66 | 5.87 | 8.69 | 9.18 | 44.50 |
Original glass | 16.18 | 2.94 | 5.88 | 8.82 | 13.24 | 52.94 |
Table 1.
Elemental atomic composition of the surface of bioactive glass particles/at%
Figure 6.XPS survey spectra of bioactive glass surface
Table 1 and Si2p spectra (Fig. 7(a)) present that an extremely weak Si signal was observed on the surface of the sample exposed to electric field. The weak signal was expected to come from the silica-riched gel layer or unreacted glass beneath the apatite layer. Hence, an apatite layer was formed on the glass surface. Additionally, for the samples exposed to electric field, O1s spectra (Fig. 7(b)) present a slight shift in peak position towards lower binding energy of 531.6 eV, which agreed well with the P-O bond of HA. Fig. 7(c) shows the strength of B1s peak increased, but symmetry decreased. This phenomenon can be related to changes in the proportion of different species, while possibly also the borate part of the network breaks down and partly dissolves.
Figure 7.XPS spectra of bioactive glass surface
As mentioned in the previously work, borosilicate glasses are composed of borate and silicate network formers, consisting of structural units such as BO3, BO4 and SiO4. BO4 and SiO4 are linked preferentially via BO3[
3 Conclusions
Electric field, as an outfield-assisted method, can assist ion exchange and diffusion in glass. In this paper, a DC electric field was applied to borosilicate bioactive glass to analyze the effect of the electric field on the degradation and in vitro mineralization property. DC electric field can increase the degradation rate of borosilicate bioactive glass, while ion dissloution can be increased significantly. In particular, the increase of boron ions can improve the bioactivity of borosilicate bioactive glasses under the premise that the mineralization property of bioactive glasses would gradually slow down with time. Meanwhile, DC electric field also can accelerate the conversion of HA, and assist glass network hydrolysis and surface hydroxylation. As an outfield-assisted approach to regulation, DC electric field can improve the mineralization performance of borosilicate bioactive glass and enhance the bioactivity. It is expected to act as an in vitro modulation to accelerate the degradation of bioactive glass and HA conversion to enhance bone repair performance.
References
[1] Q FU, N RAHAMAN M, L FU H et al. Silicate, borosilicate, and borate bioactive glass scaffolds with controllable degradation rate for bone tissue engineering applications. I.preparation and
[6] G GABRIEL F D, K LAETITIA, G YSIA I et al. Bone substitutes: a review of their characteristics, clinical use, and perspectives for large bone defects management. Journal of Tissue Engineering, 9, 1-18(2018).
[14] D LI Y, N RAHAMAN M, S BAL B et al. Conversion of bioactive borosilicate glass to multilayered hydroxyapatite in dilute phosphate solution. Journal of the American Ceramic Society, 90, 3804-3810(2007).
[20] H YAO A, J LIN, H HUANG W et al. Formation mechanism of multilayered structure on surface of bioactive borosilicate glass. Chinese Journal of Inorganic Chemistry, 24, 1132-1136(2008).
[24] S BRAUER D, D MÖNCKE. Introduction to the structure of silicate, phosphate and borate glasses. RSC Smart Materials, 23, 61-88(2016).
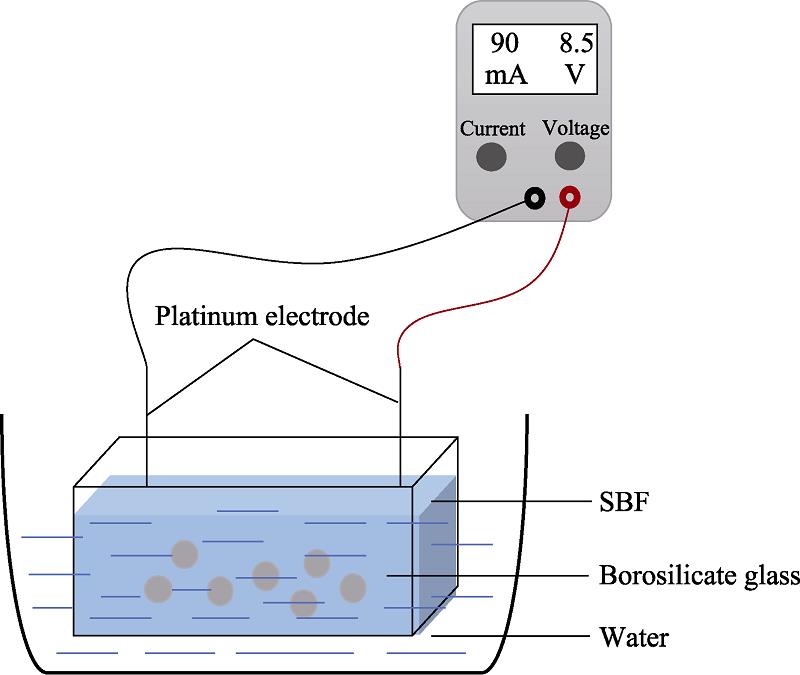
Set citation alerts for the article
Please enter your email address