Abstract
A tunable single-longitudinal-mode (SLM) semiconductor optical amplifier (SOA)-based fiber laser based on a dispersion-shifted fiber (DSF) is proposed and successfully demonstrated. SLM operation is obtained due to the spectral narrowing effect resulting from inverse four-wave mixing in a DSF. A tunable optical filter performs wavelength selection function. By inserting a length of DSF in the laser cavity, SLM lasing can possibly be obtained when laser oscillation is stably established after traveling through the DSF many roundtrips. Stable tunable SLM oscillation with a signal-to-noise ratio as high as 65 dB over a wavelength range of about 35 nm is achieved experimentally, and each spectral linewidth is less than 6.5 kHz.Single-longitudinal-mode (SLM) lasers have attracted much interest due to applications in an optical fiber sensor system[1–3], optical communications system[4], microwave generation[5], high-resolution spectroscopy optical frequency standard[6,7], laser gyroscope[8], LIDAR[9], etc. Various technologies have been proposed and demonstrated to obtain SLM operation in fiber lasers, such as injection locking[10–12], utilizing special fiber Bragg gratings (FBGs) to build a distributed Bragg reflector (DBR) or distributed feedback (DFB) fiber lasers[13–16], employing an unpumped erbium-doped fiber (EDF) or two-dimensional (2D) material, as a saturable absorber (SA)[17–20], compound cavity[21,22], or a combination of the above methods. However, most of the techniques have difficulty in achieving wide wavelength tuning, and some approaches have poor stability.
Recently, Turitsyn et al. presented a new nonlinear self-action effect, self-parametric amplification (SPA), which manifests itself as optical spectrum narrowing in a normal dispersion fiber[23]. The narrowing results from inverse four-wave mixing (FWM), resembling an effective parametric amplification of the central part of the spectrum by energy transfer from the spectral tails. Based on the effect, the spectral width of a multi-longitudinal-mode (MLM) signal can become narrower after propagation in a sufficient length of normal dispersion fiber. In this Letter, we successfully applied the spectral narrowing effect in a tunable SLM semiconductor optical amplifier (SOA)-based fiber laser using a dispersion-shifted fiber (DSF). The DSF can provide normal dispersion and can narrow the injected MLM light linewidth due to the spectral narrowing effect resulting from inverse FWM. Consequently, SLM output can possibly be obtained when laser oscillation is stably established after traveling through the DSF many roundtrips. By the adjustment of a tunable optical fiber (TOF) in the laser cavity, SLM output has been demonstrated experimentally with a tuning wavelength range of about 35 nm. The results show the linewidth of each wavelength is less than 7 kHz, and the signal-to-noise ratio (SNR) is larger than 60 dB.
The configuration of the proposed SLM SOA-based fiber laser is shown schematically in Fig. 1. It has a ring structure consisting of an SOA, two polarization controllers (PCs), a TOF, two isolators (ISOs), a 5 km length of DSF, and a 50:50 output coupler. The SOA acts as a gain medium, which has a gain of about 25 dB over a 65 nm bandwidth. The center wavelength of the TOF can be tunable with a tunable wavelength range from 1530 to 1600 nm, maintaining a 3 dB bandwidth of about 0.2 nm. The two PCs are connected before the SOA and the DSF for optimum gain performance and inverse FWM efficiency, respectively. The ISOs in the cavity are used to ensure unidirectional operation. The 5 km length of DSF is used to compress the laser linewidth. The laser output exists through the output port of the optical coupler. The laser output is further divided into two parts by another 50:50 optical coupler with one sent to an optical spectrum analyzer (OSA) with a resolution of 0.02 nm to monitor the optical spectrum, and the other is sent to a photo-detector (PD) followed by an electronic spectrum analyzer (ESA) to monitor the electrical spectrum.
Sign up for Chinese Optics Letters TOC. Get the latest issue of Chinese Optics Letters delivered right to you!Sign up now
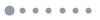
Figure 1.Schematic configuration of the proposed SLM SOA-based fiber laser.
The DSF is the key component in the laser cavity, which is used to ensure SLM lasing. As presented in Ref. [23], the spectral narrowing effect resulted from inverse FWM may occur in a fiber with normal dispersion. Consequently, an MLM light wave will have a narrower linewidth with fewer longitudinal modes after passing through the DSF, since the DSF has normal dispersion in a wavelength band shorter than 1575 nm. Therefore, SLM operation can possibly be obtained when stable laser oscillation is established after many roundtrips through the DSF in the cavity. In addition, the spectrum narrowing effect exists in the DSF for a wide wavelength range, which can be used to achieve wavelength tuning by an adjustment of the center wavelength of the TOF in the laser cavity.
In the experiment, by the adjustment of the PCs and the TOF, tunable single frequency output with a wavelength ranging from 1534.96 to 1570.02 nm has been achieved. Figure 2 shows the spectra for the whole wavelength tuning range. To verify that the laser is under SLM operation, the beating radio frequency (RF) spectrum of the laser output was measured using an ESA. One detailed spectrum at the wavelength of 1545.10 nm is depicted in Fig. 3(a), and the corresponding RF spectrum is shown in Fig. 3(b). Since the longitudinal mode spacing is estimated to be about 49 kHz, the RF spectrum confirms that there are no beating signals between longitudinal modes, and the laser was indeed under SLM operation.
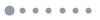
Figure 2.(Color online) Spectra of the laser output with wavelength ranging from 1534.96 to 1570.02 nm.
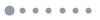
Figure 3.(a) Optical spectrum and (b) RF spectrum of the laser output.
The output spectrum linewidth was measured using the self-heterodyne method with a length of 40 km delayed single mode fiber (SMF) and an acoustic optical modulator (AOM) of 210 MHz frequency shifting. A typical delayed self-heterodyne RF spectrum (the black line) and the corresponding Lorentz curve fitting (the red line) are shown in Fig. 4. The bandwidth is about 38.9 kHz when we assume the laser spectrum to be Lorentzian-shaped, then the full width at half-maximum (FWHM) of the laser would be calculated to be about 6.48 kHz.
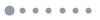
Figure 4.(Color online) Delayed self-heterodyne RF spectrum of the laser output.
As a comparison, we also measured the RF spectrum at the output of the SOA-based fiber laser when the DSF in the laser cavity was replaced by an SMF with the same length. As can be seen in Fig. 5, beat notes at about 48.5 kHz and its multiples are observed, which are resulted from the beating between longitudinal modes. It confirms that the SOA-based fiber laser is operating in the MLM status with an SMF in the laser cavity. Thus, the effectiveness of the use of DSF to achieve SLM operation is verified.
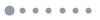
Figure 5.Beat notes observed by measuring the RF spectrum at the output of the laser when DSF was replaced with SMF.
Note that in the experiment, we used three different lengths of DSF (1.3, 3.6, and 5 km DSF), and the 5 km DSF gave the best result. This could be explained from the inverse FWM effect as discussed in Ref. [23]. As stated in Ref. [23], spectral narrowing occurs when the dispersion length becomes comparable with the nonlinear length. Consequently, there exists an optimum length of DSF for obtaining the high spectral narrowing effect. Theoretical study may be carried out, and the length selection can be provided by simulation, which would be a future work and not included in this Letter.
Another point we have to note is that an SOA instead of an EDF amplifier (EDFA) is used as the gain medium, since it can greatly shorten the laser cavity and, consequently, increase the longitudinal-mode spacing, which makes it easier to obtain the SLM operation.
In conclusion, a tunable SLM SOA-based fiber laser based on a DSF is proposed and successfully demonstrated. The spectral narrowing effect that results from an inverse FWM in the DSF is verified experimentally. The use of the DSF in a fiber ring laser to achieve SLM lasing is then demonstrated. The results show stable SLM oscillation with an SNR as high as 65 dB and a spectral linewidth of less than 7 kHz over a tuning wavelength range of about 35 nm. The proposed SOA-based fiber laser may offer potential applications in optical measurement and optical telecommunications due to its simple configuration and wide tunability.
References
[1] E. M. Strzelecki, D. A. Cohen, L. A. Coldren. J. Lightwave Technol., 6, 1610(1988).
[2] Y. Liu, M. J. Zhang, P. Wang, L. Li, Y. C. Wang, X. Y. Bao. IEEE Photon. J., 7, 6802809(2015).
[3] T. Yang, Y. Song, W. Zhang, F. Li. Chin. Opt. Lett., 14, 120602(2016).
[4] L. B. Du, A. J. Lowery. Opt. Express, 18, 17075(2010).
[5] W. Z. Li, F. Q. Kong, J. P. Yao. J. Lightwave Technol., 32, 3572(2014).
[6] M. V. Okhapkin, M. N. Skvortsov, A. M. Belkin, N. L. Kvashnin, S. N. Bagayev. Opt. Commun., 203, 359(2002).
[7] N. Dudovich, D. Oron, Y. Sillerberg. Nature, 418, 512(2002).
[8] A. Mignot, G. Feugnet, S. Schwartz, I. Sagnes, A. Garnache, C. Fabre, J. P. Pocholle. Opt. Lett., 34, 97(2009).
[9] Y. Liu, J. Liu, W. Chen. Chin. Opt. Lett., 9, 090604(2011).
[10] H. D. Wan, Z. W. Wu, X. H. Sun. Opt. Laser Technol., 48, 167(2013).
[11] C. H. Yeh, C. W. Chow. Opt. Fiber Technol., 16, 271(2010).
[12] A. Q. Zhang, X. H. Feng, M. G. Wan, Z. H. Li, B. O. Guan. Opt. Express, 21, 12874(2013).
[13] Z. Y. Dai, J. F. Li, X. X. Zhang, Z. H. Ou, Y. Z. Liu. Opt. Quantum Electron., 41, 1033(2009).
[14] H. Z. Lin, L. N. Ma, Y. M. Hu, Z. L. Hu, Q. Yao. Opt. Lasers Eng., 51, 822(2013).
[15] S. D. Lim, J. K. Yoo, S. K. Kim. Proceedings of CLEO: Science and Innovations(2016).
[16] S. C. Feng, S. H. Lu, W. J. Peng, Q. Li, T. Feng, S. S. Jian. Opt. Laser Technol., 47, 102(2013).
[17] C. H. Yeh, N. Tsai, Y. H. Zhuang, C. W. Chow, J. H. Chen. Opt. Laser Technol., 88, 180(2017).
[18] J. K. Yoo, S. D. Lim, S. K. Kim. Proceedings of IEEE Lasers and Electro-Optics(2015).
[19] S. Q. Chen, Q. K. Wang, C. J. Zhao, Y. Li, H. Zhang, S. C. Wen. J. Lightwave Technol., 32, 4438(2014).
[20] B. L. Lu, L. M. Yuan, X. Y. Qi, L. Hou, B. Sun, P. Fu, J. T. Bai. Chin. Opt. Lett., 14, 071404–1(2016).
[21] H. D. Wan, J. Wang. Proceedings of IEEE European Conference on Optical Communication(2014).
[22] C. H. Yeh, J. Y. Chen, H. Z. Chen, C. W. Chow. Opt. Laser Technol., 82, 72(2016).
[23] S. K. Turitsyn, A. E. Bednyakova, M. P. Fedoruk, S. B. Papernyi, W. R. L. Clements. Nat. Photon., 9, 608(2011).