Wunan Li, Yu Cao, Yu Ning, Fengjie Xi, Quan Sun, Xiaojun Xu, "Single-pixel wavefront sensing via vectorial polarization modulation [Invited]," Chin. Opt. Lett. 21, 090008 (2023)

Search by keywords or author
- Chinese Optics Letters
- Vol. 21, Issue 9, 090008 (2023)

Fig. 1. Vectorial polarization modulation process. The section on the left shows the polarization distribution of incident light, while the section on the right shows the polarization distribution of emergent light. Additionally, the yellow beam simulates the scenario where the light has been focused by different subapertures of the MLA.

Fig. 2. Magnified images of four typical positions. The light spot in Fig. 2(a) is located at the VR’s center, while the light spot in Fig. 2(d) is infinitely far from the VR’s center, which, in reality, is impossible. Figs. 2(b) and 2(c) are the intermediate states between the center and infinity, with the light spot in Fig. 2(b) being closer to the VR’s center.
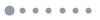
Fig. 3. Numerical simulation of a vectorial polarization distribution. Since the simulation is in an ideal situation, the angles of polarization change homogeneously, and the boundary between 0° and 180° is very clear, which is difficult to observe in real experiments.
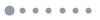
Fig. 4. Simulation results of OPD and RPD. The value range of RPD has been significantly improved compared with OPD; even the areas selected from the cell edge can be distinguished from each other.
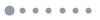
Fig. 5. Nine simulated light spots. Every pixel is endowed with an independent polarization state, forming a unique set of OPA and RPD.
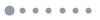
Fig. 6. Comparison of decoding errors between OPD and RPD.
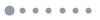
Fig. 7. Polarization centroid decoding error for different radii.
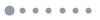
Fig. 8. (a) Experimental setup. BE, beam expander; BS, beam splitter; SLM, spatial light modulator; ND, neutral density filter; LP, linear polarizer; MLA, microlens array; VR, vortex retarder; RL, relay lens; PC, polarization camera; SP, square pinhole; SD, single-pixel detector; TS, translation stage. (b) SD installed with SP. (c) FLIR polarization camera.
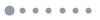
Fig. 9. Wavefront reconstruction results of the spherical wave. (a) Vectorial polarization distribution; (b) polarization spot array without wavefront distortion; (c) polarization spot array under the spherical wave; (d) phase map of the spherical wave; (e) reconstructed wavefront; (f) residual wavefront.
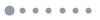
Fig. 10. Wavefront reconstruction results of complex distortion. The incident wavefront is generated randomly, with different colors within the figures representing distinct phase values.
|
Table 1. Common Parameters for Numerical Simulations

Set citation alerts for the article
Please enter your email address