
- Photonics Research
- Vol. 9, Issue 10, 1979 (2021)
Abstract
1. INTRODUCTION
Optoelectronics is one of the cornerstone technologies that promoted the rapid development of modern society’s new and smart infrastructure. In the past few decades, optoelectronic devices have entered into the field of consumer electronic products and information industry on a large scale, thereby changing the lifestyle of the masses. One such next-generation device—transparent quantum-dot light-emitting diodes (Tr-QLEDs)—have attracted extensive attention as a potential candidate for transparent display technology owing to their unique characteristics such as size-dependent tunable wavelength emission, narrow linewidth, high color saturation, high electroluminescence (EL) efficiency, high stability, and solution-processed fabrication [1–5]. Traditional LEDs, including QLEDs, organic LEDs (OLEDs), and perovskite LEDs, are opaque because their top electrodes [6] are fabricated with highly reflective metal thin films (Al, Cu, Ag, or Au). Since the first report on QLEDs in 1994 [7], considerable advances have been made in the device performance; especially the stability, lifetime, color saturation, and EL efficiency of the QLEDs have become comparable to those of the OLEDs [2]. However, despite the remarkable progress made in improving the QLED performance, the performance of the transparent LEDs is still lower than that of the nontransparent LEDs, owing to imbalanced carrier injection, energy-level mismatch, damage due to plasma sputtering, and poor conductivity of the top transparent electrodes [8–11].
In the past decade, several studies were conducted to identify plausible methods to improve the performance of Tr-QLEDs. For instance, Kim and co-workers first reported a semitransparent inverted QLED fabricated using a red quantum-dot emissive layer (QD EML). This device exhibited a maximum luminance of
Compared to the inverted Tr-QLEDs, the conventional Tr-QLEDs tend to exhibit better EL characteristics. For example, Wang and co-workers developed a highly transparent QLED using thick ZnO nanocrystals to prepare the buffer layer and electron transport layer (ETL) [13]. The resulting Tr-QLED with a high average transparency of 70% exhibited an external quantum efficiency (EQE) of 5% (current efficiency of 7 cd/A), which was comparable to that of the devices with conventional Al electrodes. In 2015, a Tr-QLED, developed using a vacuum-free fabrication process and containing an Ag NW cathode, was demonstrated. This device exhibited a reported luminance and current efficiency of over
Sign up for Photonics Research TOC. Get the latest issue of Photonics Research delivered right to you!Sign up now
Herein, we introduce efficient ZnO/ZnMgO inorganic double ETLs to achieve Tr-QLEDs with highly improved EL characteristics. In the previously reported studies, organic/inorganic double ETLs [17], double metal oxide (
2. EXPERIMENT
For this experiment, the red CdSe/ZnS QDs were purchased from Suzhou Xingshuo Nanotech Co., Ltd., and the ZnO and ZnMgO nanoparticles were procured from Guangdong Poly Opto-Electronics Co., Ltd. The organic semiconductors, including poly(3,4-ethylenedioxythiophene):poly(styrenesulfonate) (PEDOT:PSS) and poly(9,9-dioctylfluorene-co-N-(4-(3-methyl-propyl))diphenylamine) (TFB), were obtained from Luminescence Technology Corp. The patterned indium tin oxide (ITO) substrates were cleaned using an ultrasonic cleaner in which first acetone, followed by ethanol, and, finally, deionized water were used to achieve a clean surface. Next, the ITO glass was UV-ozone treated for 20 min. PEDOT:PSS (Baytron PVP Al 4083; filtered through a 0.45 mm filter) was spin coated onto the cleaned ITO glass at 3000 r/min for 45 s, and the coated glass was baked at 130°C for 15 min. Next, a layer of TFB (dissolved in chlorobenzene; 8 mg/mL) was spin coated on the previously deposited PEDOT:PSS layer at 4000 r/min for 45 s and subsequently baked at 120°C for 10 min. Third, a layer of the red QDs (dissolved in octane; 13 mg/mL) was spin coated at 4000 r/min for 45 s and baked at 100°C for 5 min. This was followed by spin coating the ZnO nanoparticles (dissolved in ethanol; 20 mg/mL) at 2000 r/min for 45 s and subsequent baking at 80°C for 20 min. After that, ZnMgO nanoparticles (dissolved in ethanol; 10 mg/mL) were spin coated, as the fifth layer, at 4000 r/min for 45 s, followed by baking at 100°C for 10 min. The thicknesses of the ZnO and ZnMgO layers were 30 and 10 nm, respectively. Finally, a top ITO electrode was deposited by magnetron sputtering at a working pressure of 0.67 Pa, power of 50 W, argon flow of 20 sccm (standard cubic centimeters per minute), and deposition time of 20 min. All the measurements were performed in air at room temperature. Details of the device characterizations are described elsewhere [20,21].
3. RESULTS AND DISCUSSION
We fabricated Tr-QLEDs, each with a structure of ITO/PEDOT:PSS/TFB/QDs/ZnO/ZnMgO/ITO, to evaluate the effect of double ETLs on the device performance. In this structure, the bottom ITO electrode, PEDOT:PSS layer, TFB layer, QD layer, ZnO/ZnMgO layers, and top ITO layer acted as the anode, hole injection layer, HTL, emission layer, double ETLs, and cathode, respectively [Fig. 1(a)].
Figure 1.(a) Structural design and (b) the corresponding energy level diagram of the Tr-QLEDs fabricated in this study [22]. Performance of the fabricated Tr-QLED device: (c) luminance–voltage (L–V) characteristics, (d) current density–voltage (J–V) characteristics, and (e) current efficiency and (f) EQE as functions of current density.
Figure 1(b) shows the energy levels of the Tr-QLEDs fabricated in this work [22]. In these Tr-QLEDs, we used TFB as the HTL because of its hole mobility (
EL Characteristics of Tr-QLEDs with Different ETLs
Peak EQE | Peak CE | Luminance | ||
---|---|---|---|---|
1 | ZnO | 9.3 | 12.6 | 34,461 |
2 | ZnO/ZnMgO | 11.8 | 16.0 | 33,764 |
CE: Current efficiency.
To examine the influence of ZnMgO on the electron injection, we fabricated an electron-only device with a structure of ITO/ZnO/QDs/ZnO with and without ZnMgO/ITO. The ZnO layer was deposited on the ITO glass to block the hole injection in this electron-only device. Figure 2(a) indicates that the current density decreased when the ZnMgO layer was added to the device. This implies that the ZnMgO layer suppressed the electron injection in the Tr-QLEDs. In the previously reported QLEDs [15,16], materials like polyvinylpyrrolidone and alumina were used to decrease the electron injection and balance the charge injection into the QDs. Figures 2(b) and 2(c) illustrate the operational mechanism of the electron-only device with and without the ZnMgO layer. When the ZnMgO layer was added to the electron-only device, the electron injection from the ITO electrode to the EML decreased because of the higher electron injection barrier between ZnMgO and ITO. This decrease in the excess injection of electrons facilitated charge balance in the device. The Tr-QLEDs with and without ZnMgO show red emission at 628 nm in Figs. 2(d)–2(g). The Tr-QLEDs with different ETLs under different voltages do not cause the wavelength to shift. The ZnMgO as modified layer restrains the electron injection and improves the charge balance in our Tr-QLEDs, but it did not lead to the Stark effect occurring under the presence of an external electric field, which could also be responsible for the redshift of the EL spectrum [30].
Figure 2.(a) Current density–voltage (J–V) curves of the electron-only device with and without ZnMgO. Energy-level diagram of the materials and the corresponding working mechanisms of the electron-only devices (b) without and (c) with ZnMgO. (d)–(g) EL spectra of the fabricated Tr-QLEDs device with and without ZnMgO driven by different voltages.
Figure 3.Bottom, top, and total emission characteristics of Tr-QLEDs without and with a ZnMgO layer: (a) and (b) luminance-voltage (L-V), (c) and (d) current efficiency, (e) and (f) EQE as a function of current density.
Figures 4(a) and 4(b) show the EL spectra and CIE
Figure 4.(a) EL spectra, (b) CIE chromaticity diagram, and (c) transmittance spectra of the fabricated Tr-QLEDs with double ETLs. The inset of (c) shows images of the device at turn-off and turn-on states. (d) Photograph of the Tr-QLED in the front of a mirror.
4. CONCLUSION
In summary, we demonstrated the fabrication of efficient Tr-QLEDs using ZnO/ZnMgO inorganic double ETLs. These double ETLs can suppress the excess injection of electrons into the EML and improve the charge balance because of the lower electron mobility of ZnMgO and higher electron injection barrier between ZnMgO and the cathode. In addition, the thick double ETLs reduce the plasma-induced damage to the device during the ITO sputtering process. As a result, the Tr-QLEDs with double ETLs exhibited a maximum EQE of 11.8% and a peak current efficiency of 16 cd/A. These values are approximately 1.27 times higher than those of the devices with pure ZnO ETL. These results show that Tr-QLEDs with double ETLs can be a potential solution for the realization and commercial production of high-performance transparent displays.
References
[1] A. Castan, H.-M. Kim, J. Jang. All-solution-processed inverted quantum-dot light-emitting diodes. ACS Appl. Mater. Interfaces, 6, 2508-2515(2014).
[2] H. Shen, Q. Gao, Y. Zhang, Y. Lin, Q. Lin, Z. Li, L. Chen, Z. Zeng, X. Li, Y. Jia, S. Wang, Z. Du, L. S. Li, Z. Zhang. Visible quantum dot light-emitting diodes with simultaneous high brightness and efficiency. Nat. Photonics, 13, 192-197(2019).
[3] L. Wang, J. Lin, Y. Hu, X. Guo, Y. Lv, Z. Tang, J. Zhao, Y. Fan, N. Zhang, Y. Wang, X. Liu. Blue quantum dot light-emitting diodes with high electroluminescent efficiency. ACS Appl. Mater. Interfaces, 9, 38755-38760(2017).
[4] P. Shen, F. Cao, H. Wang, B. Wei, F. Wang, X. W. Sun, X. Yang. Solution-processed double-junction quantum-dot light-emitting diodes with an EQE of over 40%. ACS Appl. Mater. Interfaces, 11, 1065-1070(2019).
[5] K. Ding, Y. Fang, S. Dong, H. Chen, B. Luo, K. Jiang, H. Gu, L. Fan, S. Liu, B. Hu, L. Wang. 24.1% external quantum efficiency of flexible quantum dot light-emitting diodes by light extraction of silver nanowire transparent electrodes. Adv. Opt. Mater., 6, 1800347(2018).
[6] L. Liu, K. Cao, S. Chen, W. Huang. Toward see-through optoelectronics: transparent light-emitting diodes and solar cells. Adv. Opt. Mater., 8, 2001122(2020).
[7] V. L. Colvin, M. C. Schlamp, A. P. Alivisatos. Light-emitting-diodes made from cadmium selenide nanocrystals and a semiconducting polymer. Nature, 370, 354-357(1994).
[8] H.-M. Kim, A. R. b. M. Yusoff, T.-W. Kim, Y.-G. Seol, H.-P. Kim, J. Jang. Semi-transparent quantum-dot light emitting diodes with an inverted structure. J. Mater. Chem. C, 2, 2259-2265(2014).
[9] J.-T. Seo, J. Han, T. Lim, K.-H. Lee, J. Hwang, H. Yang, S. Ju. Fully transparent quantum dot light-emitting diode integrated with graphene anode and cathode. ACS Nano, 8, 12476-12482(2014).
[10] H. Y. Kim, Y. J. Park, J. Kim, C. J. Han, J. Lee, Y. Kim, T. Greco, C. Ippen, A. Wedel, B.-K. Ju, M. S. Oh. Transparent InP quantum dot light-emitting diodes with ZrO2 electron transport layer and indium zinc oxide top electrode. Adv. Funct. Mater., 26, 3454-3461(2016).
[11] L. Yao, X. Fang, W. Gu, W. Zhai, Y. Wan, X. Xie, W. Xu, X. Pi, G. Ran, G. Qin. Fully transparent quantum dot light-emitting diode with a laminated top graphene anode. ACS Appl. Mater. Interfaces, 9, 24005-24010(2017).
[12] X. Jiang, Z. Song, G. Liu, Y. Ma, A. Wang, Y. Guo, Z. Du. AgNWs/AZO composite electrode for transparent inverted ZnCdSeS/ZnS quantum dot light-emitting diodes. Nanotechnology, 31, 055201(2020).
[13] W. Wang, H. Peng, S. Chen. Highly transparent quantum-dot light-emitting diodes with sputtered indium-tin-oxide electrodes. J. Mater. Chem. C, 4, 1838-1841(2016).
[14] P. Jing, W. Ji, Q. Zeng, D. Li, S. Qu, J. Wang, D. Zhang. Vacuum-free transparent quantum dot light-emitting diodes with silver nanowire cathode. Sci. Rep., 5, 12499(2015).
[15] M. K. Choi, J. Yang, D. C. Kim, Z. Dai, J. Kim, H. Seung, V. S. Kale, S. J. Sung, C. R. Park, N. Lu, T. Hyeon, D.-H. Kim. Extremely vivid, highly transparent, and ultrathin quantum dot light-emitting diodes. Adv. Mater., 30, 1703279(2018).
[16] H. Zhang, S. Chen. An ZnMgO: PVP inorganic-organic hybrid electron transport layer: towards efficient bottom-emission and transparent quantum dot light-emitting diodes. J. Mater. Chem. C, 7, 2291-2298(2019).
[17] M. Park, J. Song, H. Jung, M. An, J. Lim, C. Lee, J. Roh, D. Lee. Improving performance of inverted blue quantum-dot light-emitting diodes by adopting organic/inorganic double electron transport layers. Phys. Status Solidi RRL, 14, 1900737(2020).
[18] M. Park, J. Roh, J. Lim, H. Lee, D. Lee. Double metal oxide electron transport layers for colloidal quantum dot light-emitting diodes. Nanomaterials, 10, 726(2020).
[19] Q. Zhang, X. B. Gu, Q. S. Zhang, J. Jiang, X. Jin, F. Li, Z. P. Chen, F. Zhao, Q. H. Li. ZnMgO:ZnO composite films for fast electron transport and high charge balance in quantum dot light emitting diodes. Opt. Mater. Express, 8, 909-918(2018).
[20] N. Zhang, S. Ding, K. Wang, Q. Lyu, W. X. Sun. Efficient transparent quantum-dot light-emitting diodes with an inverted architecture. Opt. Mater. Express, 11, 2145-2152(2021).
[21] Z. Ren, J. Yu, Z. Qin, J. Wang, J. Sun, C. C. S. Chan, S. Ding, K. Wang, R. Chen, K. S. Wong, X. Lu, W.-J. Yin, W. C. H. Choy. High-performance blue perovskite light-emitting diodes enabled by efficient energy transfer between coupled quasi-2D perovskite layers. Adv. Mater., 33, 2005570(2021).
[22] Y. Sun, Y. Jiang, H. Peng, J. Wei, S. Zhang, S. Chen. Efficient quantum dot light-emitting diodes with a Zn0.85Mg0.15O interfacial modification layer. Nanoscale, 9, 8962-8969(2017).
[23] Y. S. Zhao, L. X. Chen, J. L. Wu, X. W. Tan, Z. H. Xiong, Y. L. Lei. Composite hole transport layer consisting of high-mobility polymer and small molecule with deep-lying HOMO level for efficient quantum dot light-emitting diodes. IEEE Electron Device Lett., 41, 80-83(2020).
[24] H. H. Fong, A. Papadimitratos, G. G. Malliaras. Nondispersive hole transport in a polyfluorene copolymer with a mobility of 0.01 cm2 V−1 s−1. Appl. Phys. Lett., 89, 172116(2006).
[25] M. D. Ho, D. Kim, N. Kim, S. M. Cho, H. Chae. Polymer and small molecule mixture for organic hole transport layers in quantum dot light-emitting diodes. ACS Appl. Mater. Interfaces, 5, 12369-12374(2013).
[26] X. Qu, N. Zhang, R. Cai, B. Kang, S. Chen, B. Xu, K. Wang, X. W. Sun. Improving blue quantum dot light-emitting diodes by a lithium fluoride interfacial layer. Appl. Phys. Lett., 114, 071101(2019).
[27] X. L. Dai, Z. X. Zhang, Y. Z. Jin, Y. Niu, H. J. Cao, X. Y. Liang, L. W. Chen, J. P. Wang, X. G. Peng. Solution-processed, high-performance light-emitting diodes based on quantum dots. Nature, 515, 96-99(2014).
[28] B. S. Mashford, M. Stevenson, Z. Popovic, C. Hamilton, Z. Q. Zhou, C. Breen, J. Steckel, V. Bulovic, M. Bawendi, S. Coe-Sullivan, P. T. Kazlas. High-efficiency quantum-dot light-emitting devices with enhanced charge injection. Nat. Photonics, 7, 407-412(2013).
[29] H. Zhang, N. Sui, X. C. Chi, Y. H. Wang, Q. H. Liu, H. Z. Zhang, W. Y. Ji. Ultrastable quantum-dot light-emitting diodes by suppression of leakage current and exciton quenching processes. ACS Appl. Mater. Interfaces, 8, 31385-31391(2016).
[30] W. D. Zhang, S. H. Ding, W. D. Zhuang, D. Wu, P. Liu, X. W. Qu, H. C. Liu, H. C. Yang, Z. H. Wu, K. Wang, X. W. Sun. InP/ZnS/ZnS core/shell blue quantum dots for efficient light-emitting diodes. Adv. Funct. Mater., 30, 2005303(2020).
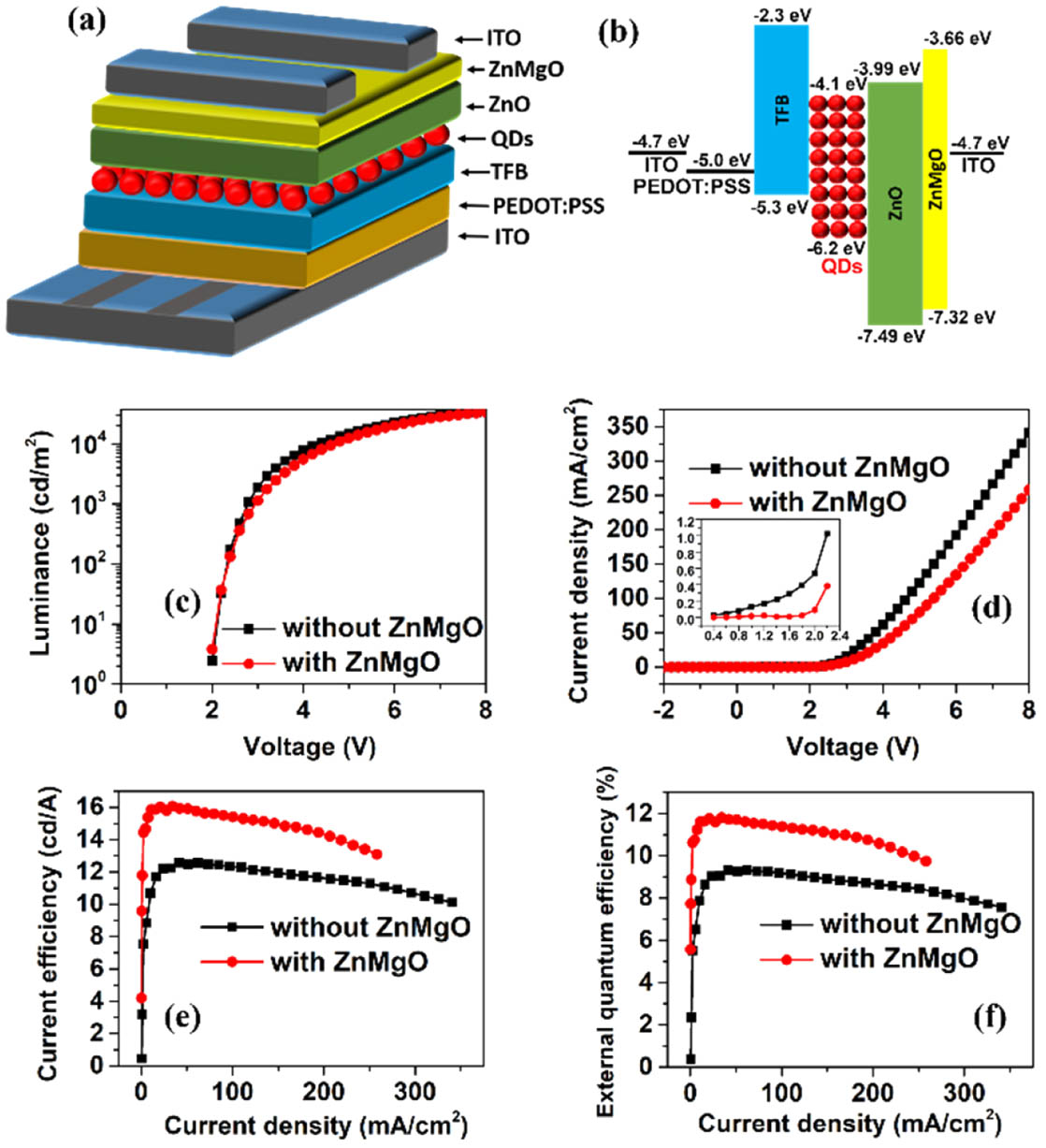
Set citation alerts for the article
Please enter your email address