Author Affiliations
1Wireless and Photonic Networks Research Center, Faculty of Engineering, University Putra Malaysia, 43400 UPM Serdang, Malaysia2Institute of Power Engineering, Universiti Tenaga Nasional, Jalan IKRAM-UNITEN, 43000 Kajang, Malaysia3Department of Physics, Kulliyyah of Science, International Islamic University Malaysia, 25710 Kuantan, Malaysia4Razak School of Engineering & Advanced Technology, Universiti Teknologi Malaysia Kuala Lumpur, Jalan Sultan Yahya Petra, 54100 Kuala Lumpur, Malaysia5Department of Communication Engineering, Faculty of Electrical and Electronics Engineering Universiti Tun Hussein Onn Malaysia, 86400 Batu Pahat, Malaysia6Department of Electrical Engineering, College of Engineering, King Saud University, Riyadh 11421, Saudi Arabiashow less
Abstract
We demonstrate a broad bandwidth multiwavelength laser based on a bidirectional Lyot filter and a semiconductor optical amplifier with a mechanism of intensity-dependent loss as the flatness agent. A wide bandwidth of a multiwavelength spectrum of 32.9 nm within a 5 dB uniformity is obtained under optimized polarization parameters. For this case, the number of generated lasing lines is 329 with a fixed wavelength separation of 0.1 nm. The power stability of this multiwavelength laser is less than 1.35 dB within 200 min time frame. This shows that the bidirectional Lyot filter provides an alternative option for multiwavelength generation in laser systems.Multiwavelength fiber lasers (MWFLs) have enticed many researchers due to their great benefit to applications such as optical communication, optical instrument testing, and optical signal processing. Gain medium based on homogeneous line broadening such as the erbium-doped fiber amplifier is usually utilized in MWFL setups[1]. However, homogeneous line broadening is renowned for its effect on strong gain competition that leads to power instability among multiwavelength lasers[2]. On the other hand, MWFLs based on a Raman amplifier[3] and a semiconductor optical amplifier (SOA)[4,5] offer inhomogeneous line broadening for a flat spectrum and a higher number of lasing lines. Between these two options, the SOA has the advantage of a simple setup and requires a low operational power as compared to the Raman amplifier. In addition, it also provides a nonlinear polarization rotation (NPR) effect that is utilized to reduce mode competition and achieve a better peak power flatness[6]. The NPR effect can induce a mechanism of either intensity dependent transmission (IDT) or intensity dependent loss (IDL) that depends on the state of polarization in the laser setup. The issue of power instability can be resolved by these techniques, IDT[1,6–8] and IDL[4,9–14], due to their function as an intensity equalizer. In order to serve this functionality, both mechanisms require a spectral filtering element in laser cavities, which can be attained by a Lyot filter configuration.
The Lyot filter has the advantage of controlling wavelength separation, simple structure, and low cavity loss[15]. The Lyot filter is also reported to have good power handling and the capability to generate narrow linewidth lasers[16]. Several works of MWFL based on a Lyot filter are realized as in Refs. [4,6,16–21]. Multiwavelength laser generation based on a unidirectional Lyot filter is limited to bandwidths of 10 nm[18,20], 15 nm[21], and 20 nm[19]. The reported values are lower than the matured wavelength division multiplexing (WDM) systems that have a 30 nm bandwidth. The main challenge of the unidirectional Lyot filter is the power uniformity between the generated laser lines. In order to rectify this problem, a bidirectional Lyot filter is proposed, as reported in Ref. [4]. However, the wavelength bandwidth is only limited to 9 nm due to the unoptimized polarization state going into the polarization beam splitter (PBS). This is due to the unequal power distribution to the bidirectional Lyot filter. In order to maximize the effect of interference in the bidirectional Lyot filter, this problem must be rectified. Therefore, the main objective of this research is to mitigate this issue by adjusting the light polarization state just before the PBS.
In this Letter, the bidirectional Lyot filter is deployed in an SOA-based fiber laser. The bandwidth of multiwavelength lasers is improved by optimizing the polarization state of light in the cavity. The bandwidth of 32.9 nm within a 5 dB power level uniformity is achieved using the proposed technique.
Sign up for Chinese Optics Letters TOC. Get the latest issue of Chinese Optics Letters delivered right to you!Sign up now
The experimental setup of MWFL with a bidirectional Lyot filter is illustrated in Fig. 1. The gain medium is SOA, manufactured by Alphion, model SAS26p. When the bias current is set to 390 mA, the peak power wavelength, peak gain, saturation output power, and polarization-dependent gain are 1490 nm, 26 dB, 9 dBm, and 1.5 dB, respectively. Meanwhile, its maximum operating bias current, active region dimensions are 600 mA and , respectively. This SOA has approximately 62 nm (1455–1517 nm) of amplified spontaneous emission (ASE) within a 5 dB bandwidth at an operating current of 550 mA. Three polarization controllers (PCs) are deployed to control the polarization state of light entering the polarization maintaining fiber (PMF) and the PBS. The bidirectional Lyot filter is constructed from the combination of PC1, PC2, and PMF as represented by the dashed box in Fig. 1.
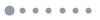
Figure 1.Schematic diagram of the MWFL setup based on the bidirectional Lyot filter.
In the proposed setup, 53.2 m long PMF with a birefringence (B) of is used as a medium of interferometry. The wavelength separation () generated by the Lyot filter is determined by , where is the operating wavelength and L is the PMF length. Another PC, which is PC3, is combined with the PBS to act as a polarizer. By adjusting the PC3, the light intensities of the PBS outputs are also changed. The PC3 is adjusted properly until the intensity of the PBS outputs are equal so that points A and B have the same intensity to obtain the optimum results. Two circulators are employed to route the light from the PBS to the bidirectional Lyot filter and from the Lyot filter to the 10/90 coupler. In our work, the bidirectional Lyot filter is used to accumulate both constructive interferences from the clockwise and counterclockwise directions and produce the effect of double interference that reshapes the interfered lights to flatten the multiwavelength spectrum. The 90% port is connected to the input port of the SOA to complete the cavity. The output is taken from the 10% port of the coupler and analyzed through an optical spectrum analyzer (OSA).
The multiwavelength generation is based on constructive interference and can be described as follows. At first, the light generated from the SOA is split into two orthogonal linear polarizations after passing through the PBS. Subsequently, the split light propagates to the bidirectional Lyot filter via circulators 1 (point A) and 2 (point B) in the clockwise and counterclockwise directions, respectively. PC1 and PC2 are employed between these two circulators to control the light polarization state before entering the PMF section. Under this condition, the light is perfectly decoupled into two orthogonal polarization axes at different speeds but with the same amplitude and polarization state. As these two waves interfere along the PMF, constructive interference occurs, creating a larger amplitude of the resultant wave than the original wave. This constructive interference effect underlines the generation of multiwavelength lasers in this work. Different PC1 and PC2 settings can lead to different angles and states of polarization, and then imperfect constructive interference can deteriorate the power uniformity of the multiwavelength laser. With proper adjustment of PC3, the intensities of light after the PBS are split equally, leading to stronger interference effects. Under this condition, the spectrum of multiwavelength lasers can be widened.
The IDL mechanism is an essential element acting as a flatness agent in producing a flat multiwavelength spectrum due to high cavity loss[22]. In this work, the IDL mechanism is induced from the combination of the SOA and polarizer[4]. In the IDL working principle, the intensity is inversely proportional to the transmission value, but proportional to the cavity loss. The strength of the IDL mechanism is reliant upon the intensity in the cavity[13]. Therefore, a lower IDL strength worsens the multiwavelength flatness as the ability in alleviating the mode competition is weaker. Thus, for this experiment, a high SOA current is needed to achieve a wide output spectrum. In this experiment, the SOA drive current is fixed to 575 mA, while all PCs are properly adjusted to achieve the optimum multiwavelength laser performance.
The effect of polarization in the proposed MWFL is investigated first. Initially, all PCs are adjusted to obtain the optimum output of the multiwavelength laser in terms of bandwidth. In this case, the bandwidth is defined as the wavelength range within a 5 dB peak power variation. When the optimized output spectrum is achieved, the angle of the half-wave plate (HWP) of PC1 is defined as 0° (initial point). Then, the HWP angle is varied to study the polarization effect on the multiwavelength output performance. Figure 2(a) represents the MWFL output at different polarization angles of HWP from 0° to 90° with a 15° interval. It can be seen that the multiwavelength output is deteriorated in terms of peak power flatness and optical signal-to-noise ratio (OSNR) for all angle values except at 0° and 90°. More details of measured bandwidth and OSNR of the multiwavelength laser at variations of the HWP rotation can be seen in Fig. 2(b). The lowest OSNR of 4 dB occurred at a polarization angle of 45°. However, this spectrum is not considered as laser as it below 5 dB uniformity. These results prove that the optimized multiwavelength is very sensitive to the light polarization state that dictates the strength of interference in the cavity. For a Lyot filter, the strongest constructive interference occurs at 45°, which is represented by the output spectrum at 0°. The same condition can be achieved for every 90° () cycle, as depicted in Fig. 2.
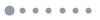
Figure 2.(a) Evolution of multiwavelength deterioration due to HWP rotation. (b) Measured bandwidth and OSNR of the multiwavelength spectrum from 0° to 90° with a 15° interval of HWP rotation.
Next, the effect of the SOA drive current on the MWFL output is investigated at the optimized polarization condition. Figure 3 shows the multiwavelength spectrum at a reduced SOA current, from 475 to 175 mA at 100 mA intervals. The measured 5 dB bandwidths at SOA currents of 475 mA, 375 mA, 275 mA, and 175 mA are 20 nm, 15.8 nm, 14.4 nm, and 8.8 nm, respectively, as illustrated in Fig. 3. The decrement of the multiwavelength bandwidth is due to the weaker IDL strength as a result of the lower light intensity circulated in the cavity[14,23]. Hence, the ability of the IDL mechanism to suppress the mode competition effectively is also degraded.
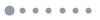
Figure 3.Degraded lasing lines and flatness due to lower SOA currents of 475, 375, 275, and 175 mA.
The impact of PC3 in the proposed MWFL is also studied. Initially, the performance of the proposed MWFL is optimized by changing all PCs to obtain the best multiwavelength performance, as depicted in Fig. 4(a). Based on this finding, the 5 dB multiwavelength bandwidth of 32.9 nm is obtained from 1547.6 nm to 1580.5 nm. The measured wavelength separation is 0.1 nm, which agrees with the calculated based on the 53.2 m long PMF, as shown in the inset of Fig. 4(a). The number of lasers generated within this bandwidth is 329 lines with 14 dB of the OSNR. The lasing lines are fairly distributed due to the intensity equalizer from the IDL mechanism. By changing PCs, the OSNR could be increased but with the trade-off of a narrower wavelength bandwidth. We have met the objective to achieve the widest wavelength bandwidth, however the maximum OSNR is only 14 dB.
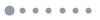
Figure 4.(a) Best multiwavelength spectrum at 575 mA of SOA current. The wavelength separation of 0.1 nm is clearly shown in the inset figure. (b) Narrower wavelength region and lower OSNR without PC3 in the setup.
In order to determine the effect without PC3 in the MWFL setup, this component is removed and the same procedure to achieve the best output is repeated, as illustrated in Fig. 4(b). From this experimental result, the 5 dB multiwavelength bandwidth is narrowed to 17.7 nm, based on the multiwavelength bandwidth from 1541.6 to 1559.3 nm, while a lower OSNR is obtained at 10 dB.
The comparison between these findings proves the importance of having PC3 in the setup to obtain wider bandwidths and higher OSNR values. This observation indicates that the property of light before entering the PBS is critical to achieving those aforementioned attributes.
The stability test is conducted for the optimized output at a 575 mA drive current and a room temperature of 25°C. The measurement is taken every 20 min in a 200 min observation period. In this experiment, three different wavelength regimes are selected, 1562.5–1563.5 nm [Fig. 5(a)], 1563.5–1564.5 nm [Fig. 5(b)], and 1564.5–1565.5 nm [Fig. 5(c)]. For each selected wavelength band, the biggest fluctuation of a laser over the time frame is chosen, as depicted in Fig. 6(a). From this analysis, the lasing wavelengths of 1563.4, 1563.7, and 1564.8 nm are selected with fluctuations of 1.35, 1.09, and 1.01 dB, respectively. The findings indicate no significant laser fluctuation in a 200 min time frame for the selected lasing wavelengths, which proves that the proposed laser is stable in terms of peak power. Figure 6(b) depicts the observation of wavelength drift in a 200 min time frame using the same lasing wavelengths as selected in Fig. 6(a). The maximum wavelength drift is 0.094 nm at lasing wavelengths of 1563.4 nm and 1564.8 nm. Meanwhile, the wavelength drift for 1563.7 nm is 0.084 nm.
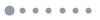
Figure 5.Measurement of power stability within 200 min in different wavelength ranges of (a) 1562.5–1563.5 nm, (b) 1563.5–1564.5 nm, and (c) 1564.5–1565.5 nm.
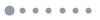
Figure 6.(a) Peak power against time for the largest deviation within the three wavelength ranges. (b) Wavelength drift observation within 200 min.
The generation of an SOA-based multiwavelength laser utilizing a bidirectional Lyot filter is demonstrated. The polarization state plays a significant role in the proposed setup to achieve the strongest constructive interference for the flattening effect. In addition, the IDL mechanism is also important to enhance the MWFL flatness and is highly dependent on the polarization state as well. The optimized multiwavelength spectrum is 32.9 nm within a 5 dB uniformity that supports 329 lasing lines with a 0.1 nm wavelength spacing. In terms of stability, the maximum peak power deviation is 1.35 dB over a 200 min observation period.
References
[1] X. Liu, L. Zhan, S. Luo, Z. Gu, J. Liu, Y. Wang, Q. Shen. Opt. Express, 20, 7088(2012).
[2] C. Yang, L. Xia, Y. Wang, D. Liu. Opt. Commun., 318, 171(2014).
[3] W. Gao, M. Liao, D. Deng, T. Cheng, T. Suzuki, Y. Ohishi. Opt. Commun., 300, 225(2013).
[4] A. H. Sulaiman, M. H. Abu Bakar, A. K. Zamzuri, S. Hitam, A. F. Abas, M. A. Mahdi. IEEE Photonics J., 5, 7101008(2013).
[5] T. Liu, D. Jia, T. Yang, Z. Wang, Y. Liu. Appl. Opt., 56, 2787(2017).
[6] A. H. Sulaiman, A. K. Zamzuri, S. Hitam, A. F. Abas, M. A. Mahdi. Opt. Commun., 291, 264(2013).
[7] Z. Zhang, J. Wu, K. Xu, X. Hong. Opt. Express, 17, 17200(2009).
[8] Z. X. Zhang, L. Zhang, Z. W. Xu. Laser Phys., 23, 1(2013).
[9] J. Tian, Y. Yao, Y. Sun, X. Yu, D. Chen. Opt. Express., 17, 15160(2009).
[10] Z. Zhang, Q. Kuang, M. Sang, Z. Ye, Y. Nie. Opt. Commun., 283, 254(2010).
[11] Z. Luo, A. Luo, W. Xu, H. Yin, J. Liu. IEEE Photonics J., 2, 571(2010).
[12] F. Li, X. Feng, H. Zheng, C. Lu, H. Y. Tam, J. N. Kutz, P. K. A. Wai. Opt. Commun., 284, 2327(2011).
[13] J. Tian, Y. Yao, J. J. Xiao, X. Yu, D. Chen. Opt. Commun., 285, 2426(2012).
[14] M. Quan, Y. Li, J. Tian, Y. Yao. Opt. Commun., 340, 63(2015).
[15] Z. Zhang, L. Zhang, Z. Xu. J. Nonlinear Opt. Phys. Mater., 21, 1250041(2012).
[16] S. Sugavanam, Z. Yan, V. Kamynin, A. S. Kurkov, L. Zhang, D. V. Churkin. Opt. Express, 22, 2839(2014).
[17] Z. X. Zhang, K. Xu, J. Wu, X. B. Hong, J. T. Lin. IEEE Photonics Technol. Lett., 20, 979(2008).
[18] Z. Zhang, L. Zhan, K. Xu, J. Wu, Y. Xia, J. Lin. Opt. Lett., 33, 324(2008).
[19] Z. X. Zhang, Z. Q. Ye, M. H. Sang, Y. Y. Nie. Laser Phys., 21, 1820(2011).
[20] X. S. Liu, L. Zhan, X. Hu, H. G. Li, Q. S. Shen, Y. X. Xia. Opt. Commun., 282, 2913(2009).
[21] S. Liu, F. Yan, F. Ting, L. Zhang, Z. Bai, W. Han, H. Zhou. IEEE Photonics Technol. Lett., 28, 864(2016).
[22] X. Feng, C. Lu, H. Y. Tam, P. K. A. Wai, D. Y. Tang, B. Guan. Opt. Laser Technol., 44, 74(2012).
[23] J. Tian, Y. Yao, J. J. Xiao, X. Xu, D. Chen. Appl. Phys. B., 102, 545(2011).