The behavior of hydrogen under extreme pressure has continued to attract great interest among the physics, chemistry, materials science, and geoscience communities and has become a major driving incentive for cross-disciplinary advances in novel high-pressure technology. This has been particularly noticeable this year, and is one of the main themes of this “Special Issue on High Pressure Science 2021.”

- Matter and Radiation at Extremes
- Vol. 7, Issue 6, 063001 (2022)
Abstract
Hydrogen exhibits a rich physics of multiple phase transitions, including a predicted novel quantum state combining superconductivity and superfluidity upon metallization, and also provides benchmark values for testing theoretical models of quantum solids at high densities. Metallic hydrogen has long been considered a holy grail of physics and astrophysics, but claims of hydrogen metallization under static compression are still under debate, mainly owing to the limited ability of presently available high-pressure techniques to unambiguously determine the transitions and metallization.1 Although superconductivity has never been realized in pure hydrogen, various hydrogen-dominant hydrides have been predicted and observed to exhibit very high-temperature superconductivity at high pressures.2 Again, reports of room-temperature superconducting hydrides are controversial owing to the difficulty of performing magnetic measurements at high pressures. In this Special Issue, Zhang et al.3 highlight the chemical aspects of the hydride bonding and structural motifs of LaH10, YH9, and CaH6 and the effects on their pressure-induced electronic properties and superconducting mechanism above 200 K. In the Earth’s deep interior, hydrogen in oxide minerals bonds with oxygen by OH bonding, symmetrical hydrogen bonding, and superionic bonding with increasing pressure and temperature. The hydrogen chemistry controls seismic, electrical, magnetic, and mechanical properties of hydrogen-bearing rocks, and dictates water transportation and circulation in the deep interior. Hu and Mao4 reassess the effects of a newly discovered superionic transition of hydrogen on our understanding of water storage and the water budget in the deep lower mantle.
As hydrogen is the lightest element, with the weakest x-ray scattering cross section, experimental studies of its properties under extreme conditions pose daunting technical challenges, and this has stimulated daring attempts to obtain definitive characterizations of structures and of physical and chemical properties at high pressures. Nuclear magnetic resonance (NMR) is one of the most sensitive techniques for probing hydrogen nuclear magnetic spins, but it has previously been impossible to use it together with high-pressure vessels. Meier et al.5 report an unprecedented advance in integrating NMR with diamond anvil cell (DAC) technology that has enabled high-resolution NMR investigations of hydrogen structures and magnetism up to multi-megabar pressures, potentially leading to breakthroughs in a number of areas of high-pressure research related to hydrogen. Another powerful probe for investigating H–H bonds, O–H bonds, and bandgap closure metallization is infrared (IR) spectroscopy, which requires a low-emittance synchrotron IR source to enable it to be applicable to the diffraction-limited small sample sizes typical of multi-megabar studies. Kong and Liu6 present a comprehensive review of state-of-the-art synchrotron-based infrared microspectroscopy (SIRMS). With its very high signal-to-noise ratio and spatial resolution, and its applicability in environments ranging from cryogenic to high temperatures, SIRMS has emerged as the workhorse for ultrahigh-pressure studies ranging from hydrous minerals in the deep Earth to hydrogen metallization. The lack of accurate pressure calibration above 200 GPa is a longstanding issue plaguing ultrahigh-pressure science. Hu and Mao7 suggest that precise, absolute pressure calibration can be obtained from micrometer-sized diamond single crystals by simultaneous x-ray diffraction (XRD) measurements of unit-cell parameters and Raman spectroscopic measurements of lattice phonons. This is based on the strict correlation between elastic moduli and the Raman-active F2g mode for diamond and provides a unique solution for this most fundamental problem of high-pressure science—quantification of the pressure variable.
The Wigner–Huntington model of metallic hydrogen, now nearly 90 years old, predicted that hydrogen would become an alkali metal with all bonding electrons delocalized at sufficiently high pressures. Recent theory and experiments, however, have found conversely that the alkali metals Li and Na are compressed into ionic solids with bonding electrons of fcc-Li and hP4-Na localized in interstices and acting as anionic interstitial quasi-atoms to form electrides. Zhang, Geng, and Wu8 report criteria that dictate anomalous longitudinal acoustic (LA)–transverse acoustic (TA) splitting of electrides.
This Special Issue also describes progress in other multidisciplinary frontier areas of high-pressure science and technology. Chen et al.9 report pressure-tuning photoluminescence of a zero-dimensional organic–inorganic hybrid metal halide (C9NH20)6Pb3Br12, a promising photonic material, with observations of robust emission over a range up to a record high pressure of 80 GPa. Wu et al.10 describe studies of pressure effects on ternary Fe–Ni–N compounds using synchrotron XRD, neutron powder diffraction, Rietveld refinement, scanning electron microscopy, and transmission electron microscopy. They have demonstrated that high-temperature and high-pressure confinement conditions favor substitutional and interstitial site disordering. Chen et al.11 present the results of an in-depth structural study on two of the most commonly used pressure media, namely, a 4:1 methanol–ethanol mixture and silicone oil up to 30 GPa. Although the media remained amorphous but became less hydrostatic, they also went through several liquid–liquid and amorphous–amorphous transitions, as characterized by XRD and Raman spectroscopic data. These results are important for understanding the structural origin of media stiffening, and they provide fundamental background information for the design of experiments using these media. Zhang et al.12 report ab initio density functional theory (DFT) simulations of the high P–T behavior of vanadium up to 600 GPa and 6000 K, which indicated a significant dependence of the temperature effects on the selection of projector augmented wave (PAW) potentials, and they also propose an optimal selection procedure for selecting PAW potentials for high P–T simulations. With such advances in high P–T techniques, a direct and accurate integrated approach to both theoretical and experimental investigations under extreme conditions is finally a realistic goal.
References
[1] P.Dalladay-Simpson, E.Gregoryanz, R. T.Howie, C.Ji, B.Li, H.-K.Mao. Everything you always wanted to know about metallic hydrogen but were afraid to ask. Matter Radiat. Extremes, 5, 038101(2020).
[2] H.Liu, J.Lv, Y.Ma, Y.Sun. Theory-orientated discovery of high-temperature superconductors in superhydrides stabilized under high pressure. Matter Radiat. Extremes, 5, 068101(2020).
[3] F.Li, G.Yang, X.Zhang, Y.Zhao. Pressure-induced hydride superconductors above 200 K. Matter Radiat. Extremes, 6, 068201(2021).
[4] Q.Hu, H.-k.Mao. Role of hydrogen and proton transportation in Earth’s deep mantle. Matter Radiat. Extremes, 6, 068101(2021).
[5] A.Aslandukova, N.Dubrovinskaia, L.Dubrovinsky, T.Ishii, S.Khandarkhaeva, D.Laniel, T.Meier, F.Trybel.
[6] L.Kong, G.Liu. Synchrotron-based infrared microspectroscopy under high pressure: An introduction. Matter Radiat. Extremes, 6, 068202(2021).
[7] Q.Hu, H.-k.Mao. Born’s valence force-field model for diamond at terapascals: Validity and implications for the primary pressure scale. Matter Radiat. Extremes, 6, 068403(2021).
[8] H. Y.Geng, Q.Wu, L.Zhang. Prediction of anomalous LA-TA splitting in electrides. Matter Radiat. Extremes, 6, 038403(2021).
[9] K.Bu, M.Chen, H.Dong, S.Guo, S.Lee, B.Liu, X.Lü, H.Luo, B.Ma, Y.Wang, Z.Yan, W.Yang. Pressure-induced robust emission in a zero-dimensional hybrid metal halide (C9NH20)6Pb3Br12. Matter Radiat. Extremes, 6, 058401(2021).
[10] L.Fang, D.He, T.Irifune, L.Lei, S.Liu, H.Ohfuji, M.Pu, Q.Tang, B.Wu, Y.Xia, F.Zhang. Pressure-induced disordering of site occupation in iron–nickel nitrides. Matter Radiat. Extremes, 6, 038401(2021).
[11] X.Chen, B.Cheng, Y.Liu, H.Lou, D.Xu, K.Yang, Q.Zeng, Z.Zeng, X.Zhang. Structural transitions of 4:1 methanol–ethanol mixture and silicone oil under high pressure. Matter Radiat. Extremes, 6, 038402(2021).
[12] S.Duan, J.Fang, X.Gao, H.Liu, H.Song, S.Wang, Y.Wang, J.Xian, T.Zhang. Effect of the projector augmented wave potentials on the simulation of thermodynamic properties of vanadium. Matter Radiat. Extremes, 6, 068401(2021).
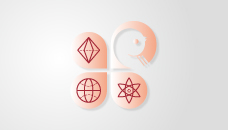
Set citation alerts for the article
Please enter your email address