S. N. Chen, F. Negoita, K. Spohr, E. d’Humières, I. Pomerantz, J. Fuchs. Extreme brightness laser-based neutron pulses as a pathway for investigating nucleosynthesis in the laboratory[J]. Matter and Radiation at Extremes, 2019, 4(5): 054402

Search by keywords or author
- Matter and Radiation at Extremes
- Vol. 4, Issue 5, 054402 (2019)
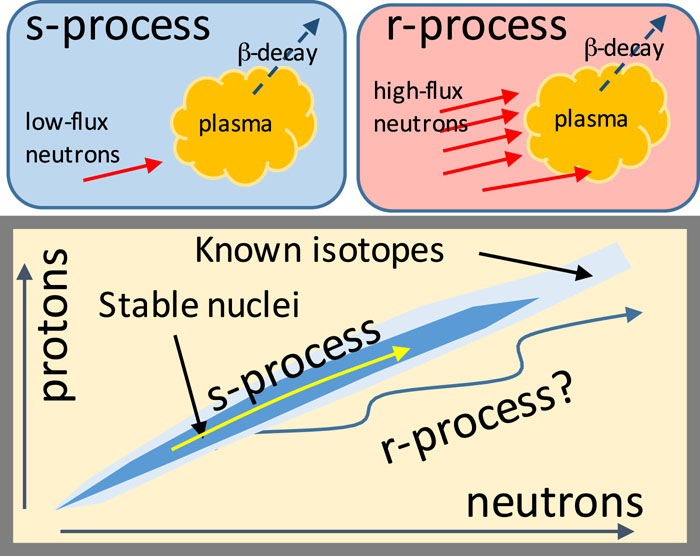
Fig. 1. Illustrations of (bottom) the domain where hadronic elements exist as a function of their proton and neutron numbers and (top) the two main processes of nucleosynthesis. The s(low)-process2 produces elements along the central domain of known elements. The r(apid)-process proceeds off-center elements through multiple neutron captures.1
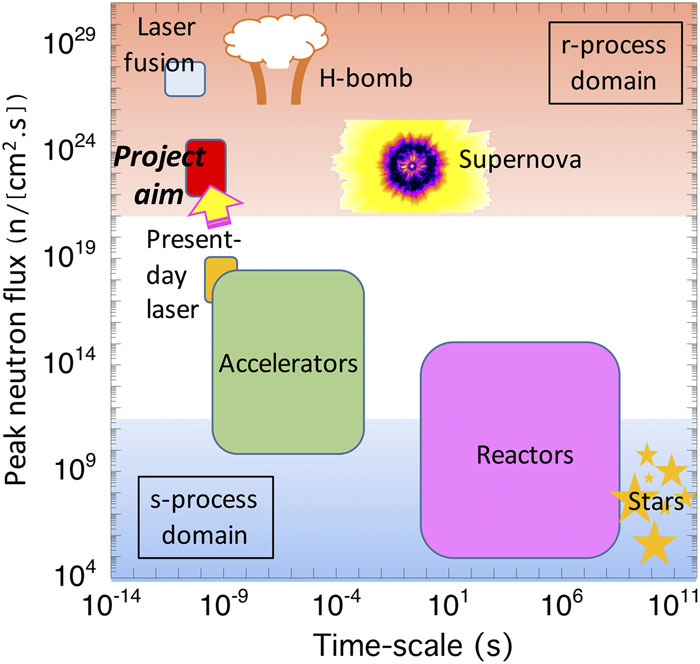
Fig. 2. Peak neutron flux vs time scale of neutron sources produced in various astrophysical sites and in facilities on Earth, showing the parameters at which the s(low)- and r(apid)-processes of nucleosynthesis take place.
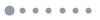
Fig. 3. Cartoon of a setup that would allow, with a multi-PW laser as a driver, the investigation of r-process nucleosynthesis in the laboratory.
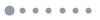
Fig. 4. Possible multi-PW laser-based platform to allow s- and r-process investigations in plasmas. The circled numbers correspond to different stages of the platform (see text).
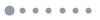
Fig. 5. Scaling of the maximum proton energy as a function of the laser intensity on target, with trendlines. Circles, squares, and diamonds are experimental points, showing that the 100 MeV threshold has been reached at today’s intensities. Crosses and stars represent simulations performed by our group using various targets (the crosses stand for RPA and the stars for SA). The yellow triangles correspond to the simulation results of Refs. 69–72 , and the brown dashed line is the theoretical scaling from Ref. 73 .
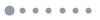
Fig. 6. PIC simulations of a wide-angle divergent (∼20° full-angle) proton beam emitted from a point source on the left and being focused as it propagates to the right. The focusing element is a cylinder of length 6 mm (its entrance being at position 0 in the figure) and diameter 700 µ m that is irradiated on its side by a laser pulse having 1020 W/cm2 intensity. Note that, for clarity, the two axes are not scaled similarly. The trajectories of 100 protons at 270 MeV are plotted, showing that the protons are well focused and collimated over long distances after the micro-lens.
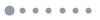
Fig. 7. Simulated neutron spectrum obtained by spallation in a 2 cm thick Pb target of a laser-driven proton beam, using the FLUKA particle transport Monte Carlo code.81 The spallation yield is 10 neutrons/proton. The dashed line represents the thermal spectrum, indicating that the spectral shape is not far from the conditions expected for the r-process.
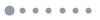
Fig. 8. Relative yields of isotopes produced by successive multi-neutron captures, i.e., by the r-process. The seed target is 96Zr. The peak neutron flux is indicated for each curve. Laser experiments are calculated for a pulse duration of 1 ns. Also shown are the relative yields produced by a 1 µ s long thermonuclear explosion.
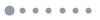
Fig. 9. Proposed setup for neutron capture measurements in hot plasmas.
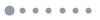
Fig. 10. Proposed setup for double-neutron capture measurements.
|
Table 1. Comparative overview of neutron beam characteristics produced at existing facilities generating the most intense neutron beams and those that will be produced by upcoming multi-PW lasers (in bold).
|
Table 2. Half-lives of 96–102Zr isotopes.
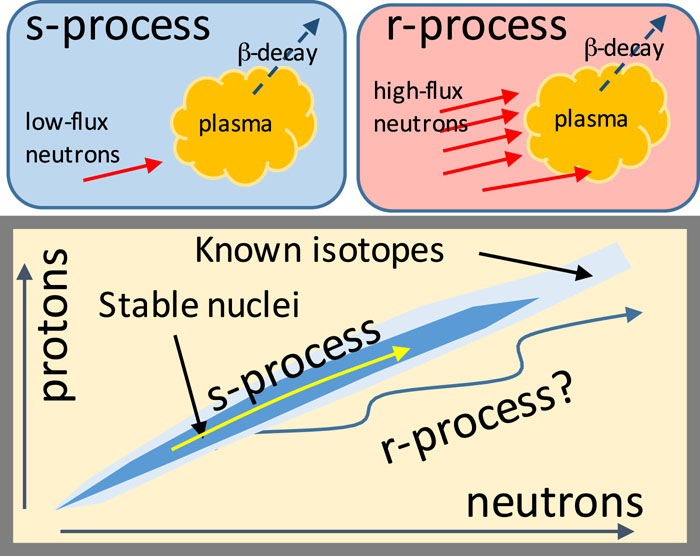
Set citation alerts for the article
Please enter your email address