Abstract
In this Letter, we demonstrate a diode-pumped electro-optical cavity-dumped Tm:YAP laser for the first time to our knowledge. A pulse width of 7.1 ns is achieved at a wavelength of 1996.9 nm. A maximum output power of 3.02 W is obtained with a pump power of 58.8 W at a repetition rate of 100 kHz and a high-voltage time of 1000 ns, corresponding to an overall optical-to-optical conversion efficiency of 5.2%. In addition, we study the effect of repetition rate and high-voltage time on the output power characteristics of a cavity-dumped Tm:YAP laser.Eye-safe, diode-pumped all-solid-state lasers operating in the spectral region near 2 μm are regarded as promising sources for various applications in Doppler wind sensing, differential absorption lidar, water vapor profiling, and low-altitude wind shear detection[1,2]. The high repetition rate, short pulse width, 2 μm pulse lasers are especially widely used in industrial processing, laser ranging, scientific research, etc.[3,4].
Compared with a Q-switched oscillator, although it could obtain a short-duration-pulse laser[5], the cavity-dumping technology has obvious advantages in many factors such as achieving constant pulse duration independent and repetition rates. In the process, the traveling time of the acoustic wave or the switching speed of the electrical signal mainly determines the pulse duration, so we can achieve the output pulse that could satisfy both a high repetition rate and a short pulse width. Compared with acousto-optical cavity-dumped lasers, the electro-optical cavity-dumped technology also has some significant advantages. For example, the electrical signal’s rising and falling edge time of the drive for the electro-optic modulator can be done very shortly, which means that we can achieve a very fast modulation speed and realize a nanoseconds trigger value, and the cavity-dumping efficiency can reach approximately a hundred percent and the pulse width of electro-optical cavity-dumped can be achieved in nanoseconds.
Diode-pumped Tm and Ho co-doped laser crystals and single Tm-doped crystals are both excellent gain media for generating a 2 μm laser[6,7]. But compared with a Ho-doped laser, a Tm-doped laser has advantages of long fluorescence lifetimes and high quantum efficiency, and the absorption peak of a Tm-doped crystal has a great amount of overlap with the emission peak of the commercial laser diode[8,9]. With the quick development of a high-power 1.9 μm laser as an efficient pump source, the 2 μm lasers based on Ho-doped[10] and Tm-doped crystals[11], such as YLF, YAG, and [12–16], have become prominent in these applications and have been known very well. The room-temperature Ho:LuAG[17–19] and Ho:ZnS have been also demonstrated. For rare-earth ion-doped laser host materials, YAP is chosen for studying as a promising efficient singly-doped laser material[20,21]. Because YAP crystallizes in the orthorhombic space group Pbnm (D162h) this low symmetry (as compared to cubic YAG) has two important consequences: the luminescence is anisotropic and the laser emission is linearly polarized[22], which has virtually no depolarization loss.
Sign up for Chinese Optics Letters TOC. Get the latest issue of Chinese Optics Letters delivered right to you!Sign up now
In this Letter, we demonstrate a diode-pumped electro-optical cavity-dumped Tm:YAP laser for the first time to our knowledge. A pulse width of 7.1 ns is achieved at a wavelength of 1996.9 nm. A maximum output power of 3.02 W is obtained with a pump power of 58.8 W at the repetition rate of 100 kHz and the high voltage time of 1000 ns, corresponding to an optical-to-optical conversion efficiency of 5.2% and a slope efficiency of approximately 9.1%. The pulses are coupled out of the cavity through a thin film polarizer (TFP) when the high voltage time is loaded on the electro-optical modulator (EOM) periodically. In addition, we study the effect of the repetition rate and the high voltage time on the output power characteristics of a cavity-dumped Tm:YAP laser.
The schematic diagram of the experimental setup is depicted in Fig. 1. The resonatorconsists of four plane mirrors and one curved mirror. The radius of curvature of the M1 is 300 mm and it is coated with 2 μm high-reflection coatings; and 45° dichroic mirrors M2 and M3 are both coated with 792 nm anti-reflection coatings and 2 μm high-reflection coatings. The plane mirror M1 is also coated with 792 nm anti-reflection coatings and 2 μm high-reflection coatings. The mirror TFP is a thin film polarizer (the incident angle is 55°) at 2.09 μm. At this time, the TFP is high-reflective () in the vertical direction and anti-reflective () in the horizontal direction for the laser. RTP is a rubidium titanyl phosphate electro-optical crystal and QWP is a quarter wave plate. The QWP can make the perpendicular linear polarization component produce a phase delay and change the state of polarization. The distances among M1-M3 are orderly 25 and 60 mm. The distances between M3 and TFP, and between TFP and M4 are 23 and 62 mm, respectively. The pump source is a fiber-coupled diode laser with a 792 nm emission wavelength. After collimating and focusing, we get the pump light spot size of 1.23 mm in diameter where it focuses in the center of the Tm:YAP crystal and, as can be seen from Fig. 1, the Tm:YAP crystal is inserted between mirrors M2 and M3. The dimensions of the laser crystal are , and it dopes 0.15 at.% Tm. Both end faces are antireflection-coated at 792 nm and 2.09 μm. The crystal is a-cut (we marked it with Pnma) along the growth direction and the crystal is fixed with the b axis in the vertical direction, so the laser polarization direction is parallel to the crystal b axis. To efficiently remove the heat generated with the pump power from the crystal, the crystal is wrapped with indium foils and held in a copper heat sink that is cooled by water. The temperature of the Tm:YAP crystal is held at . The RTP crystal is 7 mm in length and in cross section, and we use two identical RTP crystals for the compensation of natural birefringence. For the 1.99 μm RTP, the quarter-wave voltage of is . The EOM is produced by Beijing Pulse Technology Co. Ltd. and the model number is E08805.
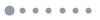
Figure 1.Experimental setup of the cavity-dumped Tm:YAP laser.
Under the condition of a 100 kHz repetition rate EOM, Fig. 2 depicts the measured results of the output power versus pump power at different high-voltage times. Because of the short lifetime of the excited state energy level of the Tm ions, to achieve a greater number of photon inversions of the upper laser level it needs longer time to accumulate. So we can see that the output power of the cavity-dumped Tm:YAP laser increases with the augmentation of the high-voltage time and the pump power. But when the pump power is over 49 W the output power becomes unstable and the linear growth trend became less smooth. We suspect that the transmittance of the RTP crystal changes periodically and, in the process of machining the length of the match for the RTP crystal, cannot reach the natural birefringent compensator. So the change of the temperature can lead to the change of transmittance of the RTP crystal, then resulting in the change of the output power. Under the high-voltage times of 500, 800, and 1000 ns in our experiment, we can get the maximum output power of 2.6, 2.7, and 3.02 W, respectively, at the biggest pump power.
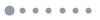
Figure 2.Output power of the cavity-dumped Tm:YAP laser versus the pump power at different high-voltage times.
Figure 3 illustrates the relationship between the measured output power of the cavity-dumped Tm:YAP laser and the frequency rate of the EOM for different pump powers and a high voltage time of 1000 ns. From Fig. 3, we can see that for low pump power the output power of the cavity-dumped laser does not change with the change in the frequency rate of the EOM. But for the high pump power the output power changes along with the frequency rate and the output power is higher while the frequency rate increases. This indicates that following the increase of the pump power the frequency rate affects the stability of the resonant cavity, and the higher frequency rate of the cavity-dumped laser is more stable than the lower frequency rate. So the results are: when the frequency rate is 50 kHz the output power changes from 0.172 to 2.42 W, and when the frequency rates are 80 and 100 kHz the output power changes from 0.205 to 2.47 W and 0.203 to 3.02 W, respectively, along with the growth of the pump power. The peak powers of the pulse laser are 425.35, 347.89, and 340.85 W, corresponding the repetition rates of 100, 80, and 50 kHz.
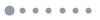
Figure 3.Output power of the cavity-dumped Tm:YAP laser versus the pump power at different repetition rates.
Figure 4 shows the typical oscilloscope trace of the pulse of the cavity-dumped Tm:YAP laser. A pulse width of 7.1 ns is obtained in the cavity-dumped Tm:YAP laser with a repetition rate of 100 kHz and a high-voltage time of 1000 ns, and the corresponding pump power is 46.5 W. The pulse width of the electro-optical cavity-dumped laser is irrelevant to the repetition rate and gain of the laser, but only associated with the length of the resonant cavity. Photons form oscillations in the cavity and, when it reaches the maximum value, the photons will be exported at the TFP by changing the polarization state. The release time is about 2L/c, where L is the optical length of the resonant cavity and c is the speed of light. When the time of the electrical signal’s falling edge is set, the pulse width of the electro-optical cavity-dumped laser is proportional to the output time of the photons.
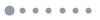
Figure 4.Output pulse profile and the pulse sequence of the cavity-dumped Tm:YAP laser.
We measure the output spectrum of the cavity-dumped Tm:YAP laser at a repetition rate of 100 kHz and a highvoltage time of 1000 ns, corresponding to the pump power of 46.5 W. The output spectrum of the cavity-dumped Tm:YAP laser is recorded by a Bristol 721A IR spectrum analyzer. The output laser spectrum is shown in Fig. 5. The emission peak is located at 1996.9 nm.
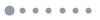
Figure 5.Output spectrum of the cavity-dumped Tm:YAP laser.
In conclusion, an electro-optical cavity-dumped Tm:YAP laser with a pulse width of 7.1 ns is achieved at a wavelength of 1996.9 nm. A maximum output power of 3.02 W is obtained with a pump power of 58.8 W, at a repetition rate of 100 kHz, and a highvoltage time of 1000 ns, corresponding to a conversion efficiency of 5.2%. It indicates that the cavity-dumped Tm:YAP laser has the potential of generating 2 μm pulses with a high power and a narrow duration.
References
[1] J. Yu, B. C. Trieu, E. A. Modlin, U. N. Singh, M. J. Kavaya, S. Chen, Y. Bai, P. J. Petzar, M. Petros. Opt. Lett., 31, 462(2006).
[2] O. A. Louchev, Y. Urata, M. Yumoto, N. Saito, S. Wada. J. Appl. Phys., 104, 033114(2008).
[3] J. L. Yang, Y. L. Tang, J. Q. Xu. Photonics Res., 1, 52(2013).
[4] V. Wulfmeyer, M. Randall, A. Brewer, R. M. Hardesty. Opt. Lett., 25, 1228(2000).
[5] D. Cao, S. F. Du, Q. J. Peng, Y. Bo, J. L. Xu, Y. D. Guo, J. Y. Zhang, D. F. Cui, Z. Y. Xu. Chin. Phys. Lett., 29, 1(2012).
[6] R. Faoro, M. Kadankov, D. Parisi, S. Veronesi, M. Tonelli, V. Petrov, U. Griebner, M. Segura, X. Mateos. Opt. Lett., 37, 1517(2012).
[7] A. Dergachev. Opt. Express, 19, 6797(2011).
[8] J. Li, S. H. Yang, H. Y. Zhang, D. X. Hu, C. M. Zhao. Laser. Phys. Lett., 7, 203(2010).
[9] Z. S. Qu, Y. G. Wang, J. Liu, L. H. Zheng, L. B. Su, J. Xu. Appl. Phys. B, 109, 143(2012).
[10] L. Han, B. Yao, X. Duan, S. Li, T. Dai, Y. Ju, Y. Wang. Chin. Opt. Lett., 12, 081401(2014).
[11] X. L. Zhang, L. Yu, S. Zhang, L. Li, J. Q. Zhao, J. H. Cui. Opt. Express, 21, 12629(2013).
[12] X. M. Duan, B. Q. Yao, Y. J. Zhang, C. W. Song, L. L. Zheng, Y. L. Ju, Y. Z. Wang. Laser Phys. Lett., 5, 347(2008).
[13] M. Schellhorn. Appl. Phys. B, 85, 549(2006).
[14] C. T. Wu, Y. L. Ju, Z. G. Wang, Q. Wang, C. W. Song, Y. Z. Wang. Laser Phys. Lett., 5, 793(2008).
[15] X. M. Duan, B. Q. Yao, C. W. Song, J. Gao, Y. Z. Wang. Laser Phys. Lett., 5, 800(2008).
[16] G. Li, Y. Gu, B. Yao, L. Shan, Y. Wang. Chin. Opt. Lett., 11, 091404(2013).
[17] X. Yang, H. Huang, D. Shen, H. Zhu, D. Tang. Chin. Opt. Lett., 12, 121405(2014).
[18] D. W. Hart, M. Jani, N. P. Barnes. Opt. Lett., 21, 728(1996).
[19] Z. Cui, B. Q. Yao, X. M. Duan, Y. Q. Du, S. Xu, Y. Z. Wang. Opt. Eng., 53, 126112(2014).
[20] R. C. Stoneman, L. Esterowitz. IEEE J. Sel. Top. Quantum Electron., 1, 78(1995).
[21] I. T. Sorokina, E. Sorokin, A. Di Lieto, M. Tonelli, R. H. Page, K. I. Schaffers. J. Opt. Soc. Am. B, 18, 926(2001).
[22] T. Y. Dai, Y. L. Ju, X. M. Duan, W. Liu, B. Q. Yao, Y. Z. Wang. Appl. Phys. B, 111, 89(2013).