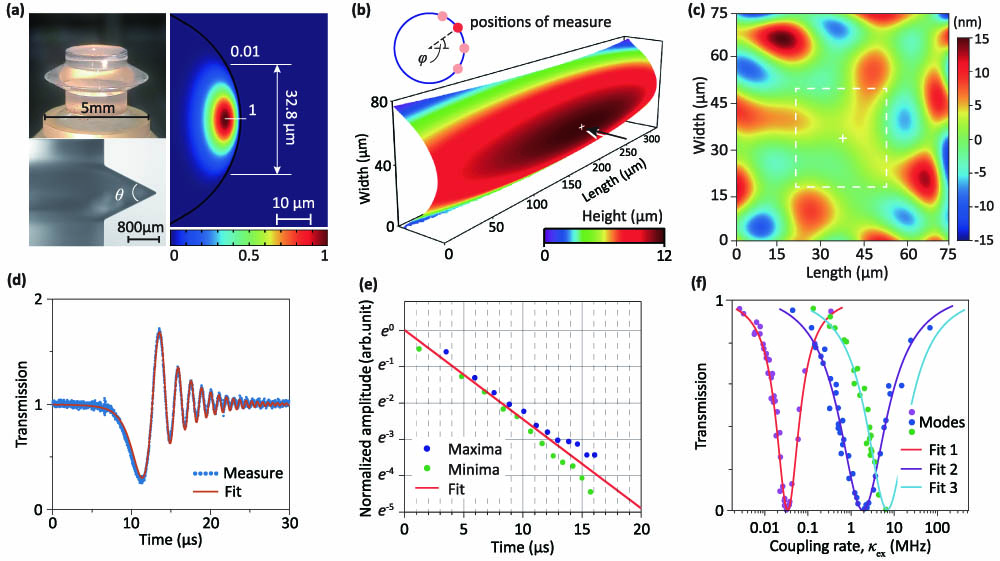
Fig. 1. Whispering gallery mode fluoride resonators. (a) Microscopic pictures of a home-made MgF2 microresonator, in which a fundamental whispering gallery mode is numerically calculated and illustrated in the normalized form. The absolute amplitude at the peak of the mode field is normalized to unity. The positions on the cavity edge where the mode field amplitude is 1% of the peak are marked, which reveals an axial range of ca. 32.8 μm covering most of the light power in the cavity. (b) A fractional 3D surface profile of the resonator, at a selected measure position on the edge. The color bar indicates the radial height of the profile. (c) The residual of the surface after being fitted and subtracted with a polynomial surface function, which in the area of 75 μm×75 μm (where the fit shows a high level of approximation) is within ±15 nm, and in 32.8 μm×32.8 μm (corresponding to the 1% mode field boundary) is within ±5 nm. The overall root mean square of the extracted residual is 3.5 nm. (d) The measured transmitted power trace of one resonance of a MgF2 resonator, which shows a ringdown profile at the ending edge upon a laser tuning speed of 350 GHz/s. Fitting of this resonance reveals: Q0=8.44×109, Qe=1.1×1010, and the loaded Q-factor is Q=4.75×109. (e) Extracted maxima and minima from the resonance trace in (a), as a function of time, which reveal a decreased linear proportion of the photon decay rate over time. The fitted photon life time (e−1 level) is 4.08 μs, corresponding to a loaded Q-factor of 4.95×109. (f) Assessment of the ideality of three resonant modes in the MgF2 resonator. The plot shows the distribution of the central transmission of these resonances, upon tuning the external coupling rate.
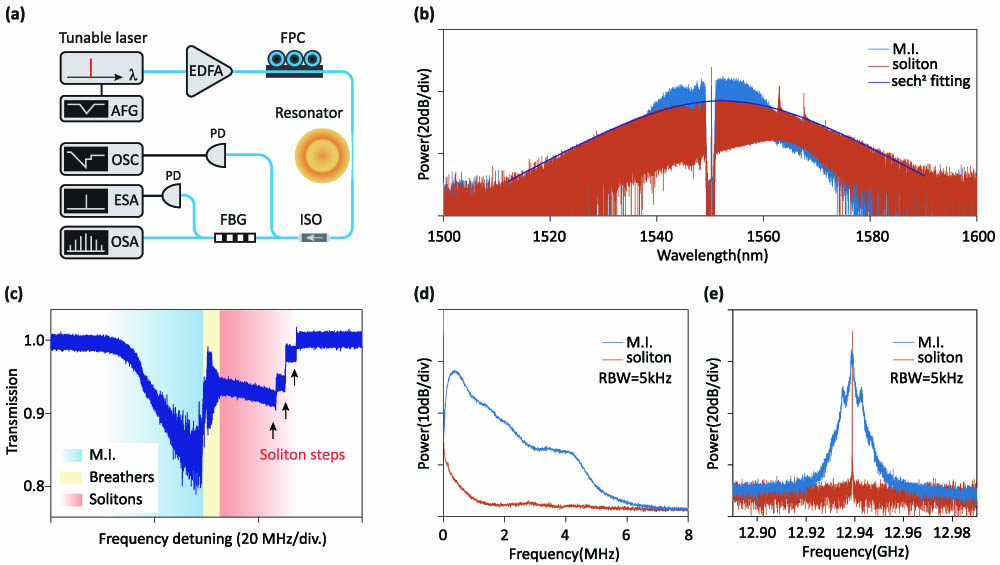
Fig. 2. Cavity Q-factors and soliton comb generation. (a) A schematic of the experimental setup. AFG, arbitrary function generator; EDFA, erbium-doped fiber amplifier; OSC, oscilloscope; ESA, electrical spectrum analyzer; OSA, optical spectrum analyzer. (b) The spectra of both an MI comb and a soliton comb. (c) The transmission of the resonance that is pumped and scanned by an intense cw laser (186.3 mW), in which the stair-like pattern (soliton steps) indicates the formation of dissipative solitons in the cavity. (d) The low-frequency RF spectrum of the filtered combs, indicating the noisy and the low-noise nature of the MI and the soliton comb states, respectively. (e) The RF spectrum around the repetition frequency of the soliton comb, which shows a narrow linewidth single-frequency tone with signal-to-noise ratio (SNR) >60 dB. The linewidth is measured to be O(10 kHz). The spectrum for the MI comb is also presented, which shows a broad line shape.
![Microcombs generated with the intermode pumping scheme. (a) Measured transmission of two resonances in proximity, probed by the laser at low power (ca. 3.8 mW). (b) The transmission of the same resonances probed at high power (ca. 281.7 mW), which reveals a soliton step. (c) Low noise RF spectrum of two combs [comb 1 and comb 2 shown in (d)] via the intermode pumping. (d) The measured comb spectra. Comb 1 is generated corresponding to the soliton step region in (b), while comb 2 is pumped regarding a second pair of resonances and is in a changed polarization state. Both combs feature intermode interactions on the central portion, and strong Raman lasing at both Stokes and anti-Stokes bands. In particular, a suspect anti-Stokes soliton comb is observed in comb 2. The full transmitted power is 238.2 mW (comb 1) and 197.3 mW (comb 2), respectively. The central comb power is estimated within the marked range (red arrows) such that the power of the Raman lasing is excluded in the calculation of power efficiency. Comb 3 is another observation that features broadband central power enhancement, and the overall spectral envelope is beyond the standard sech2-profile (compared with spectral fittings on both the central portion and on the sideband of the comb). As a consequence, the power efficiency of this comb state is also increased (pumping power 192 mW and transmitted comb power 133 mW).](/Images/icon/loading.gif)
Fig. 3. Microcombs generated with the intermode pumping scheme. (a) Measured transmission of two resonances in proximity, probed by the laser at low power (ca. 3.8 mW). (b) The transmission of the same resonances probed at high power (ca. 281.7 mW), which reveals a soliton step. (c) Low noise RF spectrum of two combs [comb 1 and comb 2 shown in (d)] via the intermode pumping. (d) The measured comb spectra. Comb 1 is generated corresponding to the soliton step region in (b), while comb 2 is pumped regarding a second pair of resonances and is in a changed polarization state. Both combs feature intermode interactions on the central portion, and strong Raman lasing at both Stokes and anti-Stokes bands. In particular, a suspect anti-Stokes soliton comb is observed in comb 2. The full transmitted power is 238.2 mW (comb 1) and 197.3 mW (comb 2), respectively. The central comb power is estimated within the marked range (red arrows) such that the power of the Raman lasing is excluded in the calculation of power efficiency. Comb 3 is another observation that features broadband central power enhancement, and the overall spectral envelope is beyond the standard sech2-profile (compared with spectral fittings on both the central portion and on the sideband of the comb). As a consequence, the power efficiency of this comb state is also increased (pumping power 192 mW and transmitted comb power 133 mW).
Fig. 4. Simulated mode map of 20 transverse mode families in magnesium fluoride resonators. For each mode family (M.F.), the resonant frequencies are compared to a uniform frequency grid ω0+μD1 (where ω0/2π and D1/2π are set as the central mode frequency and the FSR of the mode family 1). Thus, the relative frequency difference among resonant modes is extracted and illustrated. In the map, six mode families are highlighted, showing three pairs of dispersion relations (marked as case-I, -II, and -III).
Fig. 5. Simulation of soliton combs with intermode interactions. (a)–(c) Dispersion profiles of two coupled mode families, in which the primary mode family (blue dots) is unchanged, and the auxiliary mode family (orange dots) shows three different profiles, corresponding to anomalous, normal, and close-to-zero dispersion with respect to the central mode (μ=0). The generated comb modes (light blue dots) at a certain detuning (δω) are reflected as a straight line in these mode maps. (d)–(f) Transmitted spectra separately from the primary and the auxiliary mode families, i.e., (1−α)|sout,p|2 (blue line) and α|sout,aux|2 (orange line), respectively, and the combined spectrum |sout|2 (light blue drop lines) revealing wave interference in between.
Granule Size (nm) | Time (min) | RMS Roughness (nm) | |
---|
1300 | 35–40 | 125–160 | | 250 | 20–30 | 12–20 | | 50 | 15–20 | 3–5 | |
|
Table 1. Polishing Slurry Granule Size, Polishing Time, and Corresponding Roughness
| Case-I | Case-II | Case-III |
---|
| | | | | | | | | | | | | | | | | | | | | | 150 mW | | | | | | a | | | | | | | | | | 0 | | a | | | | | | | 0 | 0 | 10 | | | | | | 0 | 0 | | | | | | | | 0 | | | | 0 | | | | | |
|
Table 2. Parameters for Numerical Simulations