
- Chinese Optics Letters
- Vol. 19, Issue 7, 071404 (2021)
Abstract
1. Introduction
The pulse laser, which can be widely used in different laser fields, has been reported in a large number of literatures[
In recent years, multi-pulsed lasers with nanosecond intervals have been used in laser-induced breakdown spectroscopy (LIBS), laser drilling, laser medical treatment, and other fields[
In this Letter, we demonstrate a three-nanosecond-equal interval sub-pulse
Sign up for Chinese Optics Letters TOC. Get the latest issue of Chinese Optics Letters delivered right to you!Sign up now
We know that high-power pulses are usually obtained by
In this paper, the method of obtaining multiple pulses by multi-step
Figure 1.Typical time sequence of the generation of three-step Q-switched pulses.
2. Materials and Methods
A three-pulse output is designed to verify the method. The experimental setup of the three-nanosecond-interval sub-pulse laser is illustrated in Fig. 2. The laser system includes a diode-pumped module, a laser resonator, an electro-optic switch, a
Figure 2.Diagram of three-nanosecond-interval sub-pulse laser.
The voltage goes down three steps (Fig. 2). Each of these steps has the same time interval (
Figure 3.Waveform of electro-optic Q-switching.
3. Experimental Results and Analysis
Firstly, the performance of traditional
Figure 4.Performance of conventional Q-switching.
Secondly, the performance of three-step
Figure 5.Three sub-pulse output sequence with different time intervals.
The pulse widths of the three pulses are measured at different time intervals, as shown in Fig. 6. The pulse width and group energy have little change with the different time intervals. The pulse width fluctuates around 24 ns, 28 ns, and 36.6 ns, respectively. The pulse group energy is about 32.5 mJ, and the optical efficiency is 10.8%. This is because the fluorescence lifetime of the gain media (YAG) is about 200 µs, and the population inversion defect of nanoseconds can be ignored. This is consistent with the previous hypothesis. Compared with the traditional
Figure 6.Pulse width and energy of the three pulses versus pulse interval.
In order to obtain approximate pulsed laser output, we can regard multi-step
According to the above relationship, the sizes of
The peak powers of the three pulses are equal, so the condition of equal peak power is the product of
Thus, the pulse width and pulse energy of each pulse can be calculated:
According to the equalization condition of peak power (360 kW), the relationship between threshold gain, initial gain, and termination gain is obtained by numerical simulation (Fig. 7). The three values of the threshold gain are 1.92 cm−1, 1.23 cm−1, and 0.75 cm−1, which are basically consistent with the losses (1.992, 1.16, and 0.74) calculated by Eq. (1) at the voltages of 1280 V, 800 V, and 0 V. The energy of the three pulses is determined by the
Figure 7.Relationship of gth and gi, gf at the peak power of 360 kW.
4. Conclusions
In summary, we presented the method of achieving multiple nanosecond-interval pulses based on multi-step
References
[1] B. R. Anderson, R. Gunawidjaja, N. Gese, H. Eilers. Two-color thermometric imaging of heterogeneous materials during pulsed laser heating. Appl. Phys. B, 126, 158(2020).
[2] J. Kou, Y. Wang, X. Liu, X. Zhang, G. Chen, X. Xu, J. Bao, K. Yang, L. Yuwen. Continuous preparation of antimony nanocrystals with near infrared photothermal property by pulsed laser ablation in liquids. Sci. Rep., 10, 15095(2020).
[3] H. C. Zhao, Y. L. Qiao, X. Du, S. J. Wang, Q. Zhang, Y. Zang. Research on the technology of paint removal for aluminum alloy by pulsed laser. Chin. J. Lasers, 48, 0302001(2021).
[4] M. Wang, F. Wang, S. Feng, C. Yu, S. Wang, Q. Zhou, L. Zhang, F. Lou, D. Chen, L. Hu. 272 W quasi-single-mode picosecond pulse laser of ytterbium-doped large-mode-area photonic crystal fiber. Chin. Opt. Lett., 17, 071401(2019).
[5] C. Wang, Q. Peng, L. Xin, J. Liu. Active–passive pulse laser based on gold nanobipyramids at 1.3 µm wavelength. Chin. Opt. Lett., 17, 020003(2019).
[6] B. B. Boiko, N. S. Petrov, V. V. Valyavko, I. M. Vashkevich. Polarizers for lasers with electrooptic Q-switches. J. Appl. Spectrosc., 8, 319(1968).
[7] C. Wang, T. X. Hong, S. T. Li, Y. J. Yu, G. Y. Jin. A highly-efficient double pass Nd:YVO4 master oscillator power amplifier. Laser Phys., 29, 095002(2019).
[8] R. I. Woodward, M. R. Majewski, N. Macadam, G. Hu, T. Albrow-Owen, T. Hasan, S. D. Jackson. Q-switched Dy:ZBLAN fiber lasers beyond 3 µm: comparison of pulse generation using acousto-optic modulation and inkjet-printed black phosphorus. Opt. Express, 27, 15032(2019).
[9] S. A. Mikhnov, V. A. Kononov, R. V. Mikhnova, L. S. Korochkin. Temporal development of pulses in lasers with passive Q-switches. J. Appl. Spectrosc., 33, 694(1980).
[10] E. Hernandez-Escobar, M. Bello-Jimenez, R. Lopez-Estopier, A. Camarillo-Aviles, O. Pottiez, M. A. Garcia-Ramirez, M. Duran-Sanchez, B. Ibarra-Escamilla, M. V. Andres. Q-switching and mode locking pulse generation from an all-fiber ring laser by intermodal acousto-optic bandpass modulation. Laser Phys., 29, 015101(2019).
[11] S. Li, Q. Wang, R. Song, F. Hou, C. Gao. Laser diode pumped high-energy single-frequency Er:YAG laser with hundreds of nanoseconds pulse duration. Chin. Opt. Lett., 18, 031401(2020).
[12] X. J. Wang, F. Y. Meng, M. Li, J. B. Wang, G. B. Gao. Nanosecond dual pulse laser controlling method. Chin. J. Lasers, 38, 0203004(2011).
[13] X.-D. Wang, A. Michalowski, F. Dausinger, J.-S. Liu. Double-pulse technique for short pulse laser drilling. Laser Tech., 33, 283(2009).
[14] X. K. Miao, W. Zhao, H. W. Zhang, W. H. Yang. Research of laser encoding method based on time-controlled pulse interval. Infrared Laser Eng., 45, 1006002(2016).
[15] X. D. Wang, A. Michalowski, D. Walter, S. Sommer, M. Kraus, J. S. Liu, F. Dausinger. Laser drilling of stainless steel with nanosecond double-pulse. Opt. Laser Technol., 41, 148(2009).
[16] C.-Z. Liu, Y.-P. Dou, L. Zhang, C.-K. Sun, Z.-Q. Hao, J.-Q. Lin. Research on the dynamics of ion debris from Sn plasma by use of dual laser pulses. Spectrosc. Spect. Anal., 35, 44(2015).
[17] D. Salehinia, M. Farahmandjou, K. Khorasani, S. Behrouzinia. The continuous control of output power of a CuBr laser by a pulsed external magnetic field. Opt. Commun., 436, 143(2019).
[18] L. Shu, Y. Li, L. Chen, J. Shi, L. Chai, C. Wang, A. B. Fedotov, A. M. Zheltikov. Quarter-cycle engineering of terahertz field waveforms. Laser Phys. Lett., 11, 085404(2014).
[19] P. B. Meng, B. Q. Yao, G. Li, Y. L. Ju, Y. Z. Wang. Comparison of RTP electro-optic Q-switch and acousto-optic Q-switch in Tm, Ho:GdVO4 laser. Laser Phys., 21, 348(2011).
[20] M. Delgado-Pinar, D. Zalvidea, A. Diez, P. Perez-Millan, M. Andres. Q-switching of an all-fiber laser by acousto-optic modulation of a fiber Bragg grating. Opt. Express, 14, 1106(2006).
[21] J. R. Negri, F. Pirzio, A. Agnesi. Passively Q-switched single-frequency Nd:YVO4 ring laser with external feedback. Opt. Express, 26, 11903(2018).
[22] C. Gordon, M. Cumbajin, G. Carpintero, E. Bente, J. Javaloyes. Absorber length optimization of on-chip colliding pulse mode-locked semiconductor laser. IEEE J. Sel. Top. Quantum Electron., 24, 1100808(2018).
[23] Q. Y. Zeng, Y. Wan, J. C. Xiong, D. Y. Zhu. Modeling the pulse shape of Q-switched lasers to account for terminal-level relaxation. Chin. Phys. B, 20, 034204(2011).
[24] S. Fujikawa, K. Furuta, K. Yasui. 28% electrical-efficiency operation of a diode-side-pumped Nd:YAG rod laser. Opt. Lett., 26, 602(2001).
[25] W. Wang, C. Fu, Z. Hu, Q. Zhao, M. Gong. Segment side-pumped Q-switched Nd:YAG laser. Appl. Opt., 51, 1765(2012).
[26] Z. X. Bai, X. Q. Gao, L. Y. Chen, J. Jiang, M. Chen, G. Li. Development of a high power Q-switched laser diode-pumped Nd:YAG master oscillator power amplifier (MOPA) laser with high repetition rate. Laser Eng., 28, 233(2014).
[27] J. J. Degnan. Theory of the optimally coupled Q-switched laser. IEEE J. Quantum Electron., 25, 214(1989).
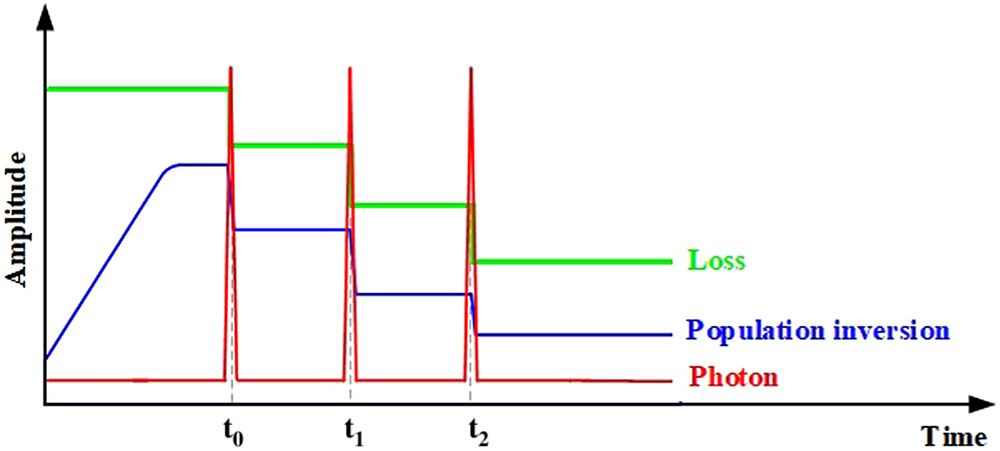
Set citation alerts for the article
Please enter your email address