Abstract
The spectral purity of fiber lasers has become a critical issue in both optical sensing and communication fields. As a result of ultra-narrow intrinsic linewidth, stimulated thermal Rayleigh scattering (STRS) has presented special potential to compress the linewidth of fiber lasers. To suppress stimulated Brillouin scattering (SBS), the most dominant disturbance for STRS in optical fibers, a semi-quantitative estimation has been established to illuminate the mechanism of suppressing SBS in a periodic tapered fiber, and it agrees with experimental results. Finally, a linewidth compression device based on STRS is integrated into a single-longitudinal-mode ring-cavity fiber laser with secondary cavities, and its linewidth is verified to be 200 Hz through a self-heterodyne detecting and Voigt fitting method.1. INTRODUCTION
Fiber lasers have been widely used in the fields of optical fiber communication, distributed optical fiber sensing, and optical spectrum analysis [1–3], where spectral purity is particularly important since it directly influences the resolution and accuracy of these applications. Mostly, spectral purity includes single-longitudinal-mode (SLM) selection and linewidth compression, and both of them have attracted more and more interest in recent research.
In order to realize SLM output, several approaches have been reported and demonstrated, such as short cavity [4,5], saturable absorber [6], twisted-mode element [7], and passive multiple ring cavities [8]. However, those conventional methods are usually influenced by the self-pulsation effect induced by interactions between doped ions in short-cavity configurations, dependence of modulation depth of the equivalent grating on pump power, and other negative factors, which leads to a linewidth broadening. Therefore, effective methods to compress linewidth are still needed. Recently, spontaneous/stimulated scattering effects in optical fibers offered an alternative method to compress the linewidth, in which the compression results were strongly dependent on the intrinsic linewidth of scattered light. Considering the intrinsic linewidth of stimulated thermal Rayleigh scattering (STRS) is on the order of kilohertz [9], STRS-based lasers have special potential in the area of ultranarrow fiber lasers. However, in optical fibers, the gain of stimulated Brillouin scattering (SBS) could be times larger than that of STRS, and increasing the power of the pump source would lead STRS to be entirely drowned in SBS, whose intrinsic linewidth is . Up to now, the main methods to suppress SBS include particular structure designs of fibers [10], phase modulation [11], and distributed parameters modulation [12–14] (such as strain, temperature, and core radius along the fiber length). Among parameter modulation methods, periodic tapered fiber is an efficient way to suppress SBS to achieve STRS-based lasers. In this paper, an SLM fiber laser with ultranarrow linewidth is demonstrated in detail, wherein passive multiple cavities are utilized to select SLM, and periodic structures of tapered fiber are employed to suppress the SBS effect in single mode fiber (SMF). To design and optimize the tapered structures, a semi-quantitative estimation has been built to illuminate the mechanism of SBS suppression with the periodic tapered fiber.
2. PRINCIPLE OF SBS SUPPRESSION METHOD
As detailed in Section 1, SBS is one of the most dominant disturbances for STRS in optical fibers, and the suppression of SBS has therefore become a major issue for STRS-based fiber lasers. In this section, a semi-quantitative estimation has been established to demonstrate the principle of the SBS suppression method based on tapered fibers. In general, SBS is regarded as an inelastic entanglement between the photons and acoustic phonons, wherein the interaction between the incident and scattered photons would modulate the material properties profoundly through the electrostriction and thermal absorbtion, and eventually influence the evolution of phonons. Such a process could be described mathematically by a set of acousto-optic coupled equations with the assumptions of the slowly varying envelope approximation and undepleted pump [15]: where , , , and are the frequencies and optical power of Stokes and pump lights, respectively; is the loss coefficient of the optical fiber; , , and are the Brillouin frequency shift (BFS), full width at half maximum (FWHM), and peak gain of SBS gain spectrum (BGS), where is mostly inversely proportional to and ; denotes the acousto-optic effective area of the fiber and could be approximately replaced by optical effective area in fibers with quasi-rectangular index profile.
Sign up for Photonics Research TOC. Get the latest issue of Photonics Research delivered right to you!Sign up now
In SBS progress, Eq. (1) has a well-defined initial condition: , which means the power of seed photons could be estimated by the Rayleigh–Jeans approximation ; and are respectively Boltzmann’s constant and the fiber temperature; and revising constant 2 is given by polarization scrambling. Equation (1) can be considered as a Cauchy problem of a first order inhomogeneous differential equation, and its general solution could be expressed as where BGS can be generally written as
Equation (3) indicates the cumulative increase of the scattered light at a certain frequency and certain location . The total generated Stokes power at the exit end () should especially be paid attention to, and it can be obtained through the integration over the whole spectrum:
It is worth indicating that cannot be described using an analytic expression because both and depend strongly on the radius of the fiber core. However, the dependences do not have definite mathematical expressions in tapered fibers with a very complex axial distribution as shown in Fig. 1. Based on Ref. [16], the relationship between and in weakly guiding acoustic waveguides can be written as where is the -order Bessel function of the first kind; is the -order modified Bessel function of the second kind; is the phase velocity of the phonons; and are the longitudinal acoustic velocities of core and cladding materials, respectively; is the refractive index of the core.
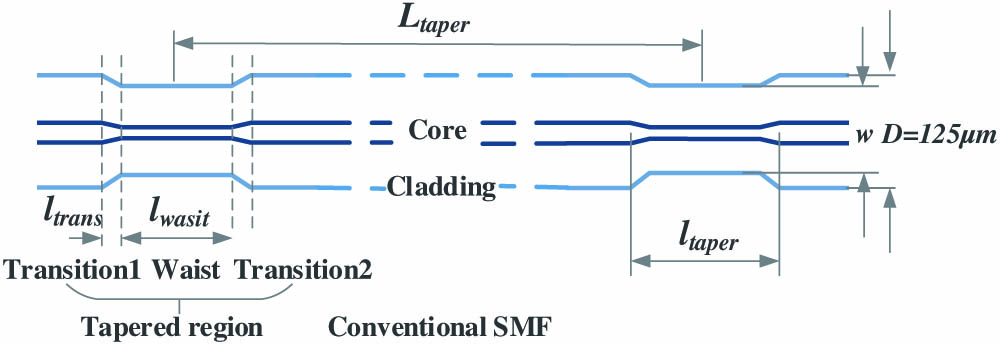
Figure 1.Typical sketch of a tapered fiber, where and are diameters of the waist and conventional SMF, respectively; the repetition length is the distance between two adjacent tapers; , , and are the lengths of the waist region, transition region, and tapered region.
Another influence caused by the tapered structure is the variation of the optical effective area. In a step-index SMF, the optical effective area can be estimated by Marcuse’s equation: where ; the numerical aperture (NA) is typically 0.12 in Corning SMF 28e fibers. Equation (6) is effective only if the fundamental mode is definitely guided by the fiber; nevertheless, the light will leak out to the air once the mode field has expanded to the air–cladding boundary. Thus, reaches its maximum when the taper waist reaches the core-mode cutoff point , which corresponds to a waist diameter of in Corning SMF28e fibers [17].
Figure 2(a) shows the numerical solutions of Eqs. (5) and (6) with common parameters of SMF (, , ). It is clear that both BFS (orange line) and (blue line) change a lot according to the variation of the radius of the fiber core, and SBS therefore gets a suppression in tapered fibers since the nonuniformly distributed BFS will restrain the global modulation caused by the incident and scattered photonics. So far, a mathematical model to describe the SBS spatial optical power evolution in a well-defined tapered fiber has been built by substituting Eqs. (5) and (6) into Eqs. (3) and (4).
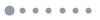
Figure 2.Simulation results: (a) and BFS versus core radius: (b) BGS versus waist, (c) total generated Stokes power versus waist, (d) BGS versus , and (e) total generated stokes power versus .
According to Burns’s parabolic model, the length ratio of the waist region to the transition region could be regarded as a function of the waist diameter [18], so that in a fixed-length segment of tapered fiber, the SBS suppression effect is relevant to only two independent variables: waist diameter and duty ratio . Figures 2(b) and 2(c) show the BGS versus different and , respectively. It is clear that both the reduction of and the increase of would decrease the amplitude of BGS, which means that SBS can be effectively suppressed in this way. Additionally, it can be seen from the comparison of Figs. 2(c) and 2(e) that BGS gain seems to be more alternative to than , and the inflection points of the curves are (75 μm, 0.58) and (0.08, 0.12), respectively. Thus, two major findings can be achieved: (1) BGS cannot be unlimitedly reduced when decreasing , and it would lead to a great loss because of the leaky mode. So the optimal is . (2) Increasing could effectively suppress SBS, and SBS is almost completely suppressed when .
3. EXPERIMENTS
Figure 3(a) shows the experimental setup to verify the validity of the SBS suppression method proposed in Section 2. A 110-m-long segment of fiber under test (FUT) is pumped by a tunable laser (TLS, Yenista Tunics-Reference, with 500-kHz linewidth) and an erbium-doped fiber amplifier (EDFA, Opeak EDFA-C-PM-CW-MB, with maximum gain of 30 dB). Via the first and second ports of the optical circulator, the pump light enters FUT, where the stimulated scattering would occur once the pump power reaches the threshold. Then, the backward-scattered light would emit from the third port of the optical circulator and be monitored by an optical spectrum analyzer (OSA, Anristu MS9740A with an FMHW resolution of 30 pm and a readout resolution of 1 pm) and an optical power meter (OPM). A variable optical attenuator (VOA) is used to restrict the power into the OSA.
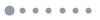
Figure 3.Experimental results of SBS suppression method: (a) experimental setup; (b)–(e) theoretical/experimental power ratio between the SBS and Rayleigh scattering peaks versus when the waist is 105, 90, 75, and 60 μm, respectively; (f) optical power spectra of backward-scattered light in conventional SMF and tapered fibers ( and ) with the same pump power.
It could be found from the OSA that, whether in conventional SMF or tapered fiber, the power spectra of backward-scattered light would split into two power peaks with central wavelengths of 1549.998 nm (contributed by Rayleigh scattering) and 1550.081 nm (contributed by SBS and BFS at ). By integrating these power peaks, the total optical power of Rayleigh and Brillouin scattering can be obtained.
To make a further verification of the proposed SBS suppression method, several tapered fibers with different and were fabricated and employed as FUTs in experiments (owing to the limitation of equipment in our laboratory, tapered fibers with are available). As indicated in Figs. 3(b)–3(e), the experimental data points show clear downward trends as the increases. It could be found from the comparison among these figures that decreasing the waists of tapers is also conducive to SBS suppression, but further decreasing the waist would not yield a proportional benefit, especially when . Meanwhile, more points with big error are observed when the waist diameter decreases to 60 μm as a result of the leak of the fundamental mode. Synthesizing the effects of SBS suppression, tapers with and are considered most practical. Figure 3(f) shows the power spectra of backward-scattered light in conventional SMFs (orange line) and tapered fibers with and (gray line) under the same condition, and a 1.84 dB decrease of total generated Stokes power is achieved in such a tapered fiber, which is close to the theoretical value of 2.37 dB.
Then, experiments are conducted to identify the component of Rayleigh power peak. STRS and spontaneous Rayleigh scattering (SponRS) cannot be directly distinguished by an OSA with a spectral resolution of 30 pm because they have nearly same frequencies and narrow linewidths ( for SponRS and for STRS). However, STRS and SponRS have linear and nonlinear responses, respectively, to the incident optical power [19]. If STRS occupies the domain part in backward scattering when the input optical power exceeds the threshold power, the power response curve should be nonlinear. So STRS and SponRS could be differentiated by drawing the response curve of the scattering optical power to incident optical power. As shown in Fig. 4, when the incident optical power increases, scattered optical power responds nonlinearly and has a threshold of 60–65 mW; therefore, there is evidence to believe STRS occurs when the optical power in the cavity is over 60–65 mW, and it would be the domain part in backward scattering.
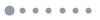
Figure 4.Variation tendency of the scattered optical power when the optical power of incident light changes.
Finally, an STRS-based fiber laser is built to further examine the performances of the tapered fiber. It is shown in Fig. 5 that the proposed laser could be simply divided into three parts: first, a fundamental multilongitudinal-mode (MLM) ring-cavity laser consisting of a 980 nm pump laser, 980/1550 nm wavelength division multiplexer (WDM), 80-cm erbium-doped fiber (EDF, Liekki Er110), fiber Bragg grating (FBG), optical circulator 1 and 90:10 coupler; second, a linewidth compression device employed to generate STRS and compress the linewidth consisting of optical circulator 2 and tapered fiber; and finally, an SLM selection device consisting of secondary cavities 1 and 2 (with cavity lengths of 0.3 and 0.5 m, respectively) made by optical couplers.
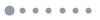
Figure 5.Schematic diagram of the tunable STRS-based SLM laser with ultranarrow linewidth.
While the laser works, the scattered light will have million-time transition trips in the ring cavity during the fluorescence relaxation time of erbium ions (), which would eventually create an ultrahigh gain contrast ratio between Rayleigh scattering and SBS, and compress the linewidth. During the experiment, an OPM is used to monitor the output power of the laser, and according to the value of the OPM and the coupling ratio of the coupler, the optical power in the ring cavity is , exceeding the threshold of STRS. So STRS would occupy the domain part in backward scattering whose gain spectrum is much narrower. Thus the tapered fiber has the potential to compress the linewidth of the fiber laser.
A self-heterodyne detection method is utilized to measure the spectrum of the STRS-based laser and, as shown in Figs. 6(a) and 6(b), MLM output with 32-MHz free spectral range and SLM output are respectively observed when secondary cavities are excluded or included in the lasers. The Voigt fitting method is also employed in our experiment to mitigate the influences caused by long delayed fiber; more details can be found in Ref. [20]. It is shown in Figs. 6(c) and 6(d) that the measured linewidth of the laser operating in SLM is , much narrower than the 9.5 kHz achieved without an SRTS-based linewidth compression device.
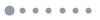
Figure 6.Spectra detected by delayed self-heterodyne method: (a) without secondary cavities, (b) with secondary cavities, (c) without linewidth compression device, and (d) with linewidth compression device.
4. CONCLUSION
In this paper, an SBS suppression method using periodic tapered fibers is proposed, and a semi-quantitative estimation is built to illuminate the mechanism of suppressing SBS and to be a guide to design an STRS-based linewidth compression device. Experimental results of BGS/total generated Stokes power verify theoretical estimations of the SBS suppression method. Based on this theory, an optimized design of a 110-m-long segment of tapered fiber with and is achieved, and total generated Stokes power declines by 1.84 dB in comparison to that in a conventional SMF, which agrees with theoretical estimations and simulations of . Due to a large ratio of fluorescence relaxation time of erbium ions to a single transition time of scattered light in the ring cavity, SBS could be effectively suppressed and linewidth compression effect of STRS could be realized. Finally, an STRS-based SLM ring-cavity fiber laser is developed wherein multiple passive cavities and periodic structures of tapered fiber are respectively utilized to select SLM and compress linewidth, and its linewidth is experimentally verified to be downward from 9.5 kHz to 200 Hz through a self-heterodyne detection and Voigt fitting method.
References
[1] T. Liu, Y. Du, Z. Ding, K. Liu, Y. Zhou, J. Jiang. 40-km OFDR-based distributed disturbance optical fiber sensor. IEEE Photon. Technol. Lett., 28, 771-774(2016).
[2] A. Bergman, R. Davidi, A. I. Shalev, L. Ovadia, T. Langer, M. Tur. Increasing the measurement dynamic range of Rayleigh-based OFDR interrogator using an amplifying add-on module. IEEE Photon. Technol. Lett., 28, 2621-2624(2016).
[3] K. Feng, J. Cui, H. Dang, S. Zhao, W. Wu, J. Tan. Investigation and development of a high spectral resolution coherent optical spectrum analysis system. Opt. Express, 24, 25389-25402(2016).
[4] C. Spiegelberg, J. Geng, Y. Hu, Y. Kaneda, S. Jiang, N. Peyghambarian. Low-noise narrow-linewidth fiber laser at 1550 nm (June 2003). J. Lightwave Technol., 22, 57-62(2004).
[5] S. Xu, Z. Yang, W. Zhang, X. Wei, Q. Qian, D. Chen, Q. Zhang, S. Shen, M. Peng, J. Qiu. 400 mW ultrashort cavity low-noise single-frequency Yb3+-doped phosphate fiber laser. Opt. Lett., 36, 3708-3710(2011).
[6] Z. Meng, G. Stewart, G. Whitenett. Stable single-mode operation of a narrow-linewidth, linearly polarized, erbium-fiber ring laser using a saturable absorber. J. Lightwave Technol., 24, 2179-2183(2006).
[7] D. Chang, M. Guy, S. Chernikov, J. Taylor, H. Kong. Single-frequency erbium fiber laser using the twisted-mode technique. Electron. Lett., 32, 1786-1787(1996).
[8] F. Yin, S. Yang, H. Chen, M. Chen, S. Xie. 60-nm-wide tunable single-longitudinal-mode ytterbium fiber laser with passive multiple-ring cavity. IEEE Photon. Technol. Lett., 23, 1658-1660(2011).
[9] T. Zhu, X. Bao, L. Chen, H. Liang, Y. Dong. Experimental study on stimulated Rayleigh scattering in optical fibers. Opt. Express, 18, 22958-22963(2010).
[10] S. R. Bickham, A. Kobyakov, S. Li. Nonlinear optical fibers with increased SBS thresholds. Optical Fiber Communication Conference, OTuA3(2006).
[11] F. Willems, W. Muys. Suppression of interferometric noise in externally modulated lightwave AM-CATV systems by phase modulation. Electron. Lett., 29, 2062-2063(1993).
[12] N. Yoshizawa, T. Imai. Stimulated Brillouin scattering suppression by means of applying strain distribution to fiber with cabling. J. Lightwave Technol., 11, 1518-1522(1993).
[13] J. Hansryd, F. Dross, M. Westlund, P. Andrekson, S. Knudsen. Increase of the SBS threshold in a short highly nonlinear fiber by applying a temperature distribution. J. Lightwave Technol., 19, 1691-1697(2001).
[14] K. Shiraki, M. Ohashi, M. Tateda. Suppression of stimulated Brillouin scattering in a fibre by changing the core radius. Electron. Lett., 31, 668-669(1995).
[15] A. Kobyakov, M. Sauer, D. Chowdhury. Stimulated Brillouin scattering in optical fibers. Adv. Opt. Photon., 2, 1-59(2010).
[16] C.-K. Jen, A. Safaai-Jazi, G. W. Farnell. Leaky modes in weakly guiding fiber acoustic waveguides. IEEE Trans. Ultrason. Ferroelectr. Freq. Control, 33, 634-643(1986).
[17] S. A. Ibrahim, N. A. M. A. Hambali, K. D. Dambul, S. Syahali. Mode power evolution in tapered single-mode fiber. International Symposium on Telecommunication Technologies (ISTT), 38-41(2012).
[18] W. K. Burns, M. Abebe, C. A. Villarruel. Parabolic model for shape of fiber taper. Appl. Opt., 24, 2753-2755(1985).
[19] R. W. Boyd. Nonlinear optics. Handbook of Laser Technology and Applications (Three-Volume Set)(2003).
[20] M. Chen, Z. Meng, J. Wang, W. Chen. Ultra-narrow linewidth measurement based on Voigt profile fitting. Opt. Express, 23, 6803-6808(2015).