
- Matter and Radiation at Extremes
- Vol. 6, Issue 1, 013001 (2021)
Abstract
Materials transform abruptly under compression, with their properties varying as strong functions of pressure. Advances in high-pressure and probe technology have enabled experimental characterizations up to several hundred gigapascal (GPa). Studies in the physical sciences are now expanding to include a vast previously uncharted pressure region in which transformative ideas and discoveries are becoming commonplace. Matter and Radiation under Extremes (MRE) is taking advantage of this opportunity to provide a forum for publishing the finest peer-reviewed research in high-pressure science and technology on the basis of its interdisciplinary interest, importance, timeliness, and surprising conclusions. This MRE HP Special Volume gathers together a set of contemporary perspectives, highlights, reviews, and research articles in multiple disciplines of high-pressure physics, chemistry, materials, and geoscience that illustrate both current and forthcoming trends in this exciting research area.
The year 2020 has turned out to be a bountiful one for high-pressure physics, culminating in milestone achievements in a couple of century-old quests: room-temperature superconductivity and metallic hydrogen. These two phenomena are closely related to each other: the new families of superconductors with the highest critical temperature Tc can also be viewed as hydrogen alloys with minor impurities of key secondary elements. Theorists at Jilin University have searched the periodic table for the most appropriate secondary elements and have predicted the specific structures and Tc of the resulting materials at high pressures, leading the way for experimentalists to successfully discover some highest-Tc superconductors based on hydrogen-dominant compounds alloyed with sulfur and rare-earth elements. In this HP Special Collection, Lv et al.
Successful scientific investigations at high pressure rely on the availability of appropriate technology. The symbiotic development of diamond anvil cells (DACs) to create ultrahigh P–T environments and synchrotron X-ray techniques to probe materials in situ has enabled the exploration of many areas of high-pressure science. Beyond the current static compression frontier, pure hydrogen has been predicted to transform to a metal that has been postulated to be a wonder material as a high-Tc superconductor, a two-component (electron and proton) superfluid, a new state of matter, the most energetic material, the most efficient fusion fuel, and the main constituent of giant planets and other celestial bodies. Attempts to reach the necessary pressures and to demonstrate metallization have become a century-old quest by physicists and astrophysicists, and have provided a unique driving force for advancing the high-pressure experimental frontier. Gregoryanz et al.
External pressure directly compresses chemical bonding and affects the structure of condensed matter, thus having a great impact on chemistry and its applications to materials sciences. Yoo
Recent progress in high-pressure geoscience is particularly exciting. For the first time, major cataclysmic events in the atmosphere, hydrosphere, biosphere, and lithosphere during Earth’s long history can be unified under a single theory based on pressure-induced chemistry in the bottom half of the lower mantle. Mao and Mao
In the future, after this debut 2020 issue, we aim to make the MRE HP Special Volume a yearly event to cover key progress in diverse areas of this multidisciplinary field.
References
[1] H. Liu, J. Lv, Y. Ma, Y. Sun. Theory-orientated discovery of high-temperature superconductors in superhydrides stabilized under high pressure. Matter Radiat. Extremes, 5, 068101(2020).
[2] X.-J. Chen, A. Gavriliuk, E. Greenberg, C. Ji, B. Li, H.-k. Mao, V. Prakapenka, V. Struzhkin, I. Troyan. Superconductivity in La and Y hydrides: Remaining questions to experiment and theory. Matter Radiat. Extremes, 5, 028201(2020).
[3] X.-J. Chen. Exploring high-temperature superconductivity in hard matter close to structural instability. Matter Radiat. Extremes, 5, 068102(2020).
[4] P. Dalladay-Simpson, E. Gregoryanz, R. T. Howie, C. Ji, B. Li, H.-K. Mao. Everything you always wanted to know about metallic hydrogen but were afraid to ask. Matter Radiat. Extremes, 5, 038101(2020).
[5] R. Ahuja, A. Björling, Y. Ding, E. Greenberg, X. Huang, C. Ji, B. Li, W. Liu, W. Luo, A. Majumdar, H.-K. Mao, W. L. Mao, Y. Meng, V. B. Prakapenka, G. Shen, J. Shu, S. Sinogeikin, J. S. Smith, A. Soldatov, J. Wang, R. Xu, W. Yang. Crystallography of low Z material at ultrahigh pressure: Case study on solid hydrogen. Matter Radiat. Extremes, 5, 038401(2020).
[6] N. Hirao, K. Hirose, S. I. Kawaguchi, Y. Ohishi, E. Ohtani, K. Shimizu. New developments in high-pressure X-ray diffraction beamline for diamond anvil cell at SPring-8. Matter Radiat. Extremes, 5, 018403(2020).
[7] J. Li, D. Walker. Castable solid pressure media for multianvil devices. Matter Radiat. Extremes, 5, 018402(2020).
[8] C.-S. Yoo. Chemistry under extreme conditions: Pressure evolution of chemical bonding and structure in dense solids. Matter Radiat. Extremes, 5, 018202(2020).
[9] Y. Tian, B. Xu. Diamond gets harder, tougher, and more deformable. Matter Radiat. Extremes, 5, 068103(2020).
[10] W. Deng, S. Guan, D. He, Q. Li, X. Li, A. Liang, Y. Su, J. Wang, J. Yang. Strength enhancement of nanocrystalline tungsten under high pressure. Matter Radiat. Extremes, 5, 058401(2020).
[11] B. Chen. Exploring nanomechanics with high pressure techniques. Matter Radiat. Extremes, 5, 068104(2020).
[12] M. Li, T. Liu, X. Lü, Y. Wang, W. Yang. Pressure responses of halide perovskites with various compositions, dimensionalities, and morphologies. Matter Radiat. Extremes, 5, 018201(2020).
[13] H.-k. Mao, W. L. Mao. Key problems of the four-dimensional Earth system. Matter Radiat. Extremes, 5, 038102(2020).
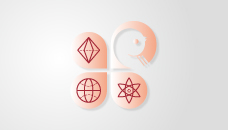
Set citation alerts for the article
Please enter your email address