Xiangjun Li, Jie Yin, Jianjun Liu, Fangzhou Shu, Tingting Lang, Xufeng Jing, Zhi Hong, "Resonant transparency of a planar anapole metamaterial at terahertz frequencies," Photonics Res. 9, 125 (2021)

Search by keywords or author
- Photonics Research
- Vol. 9, Issue 2, 125 (2021)
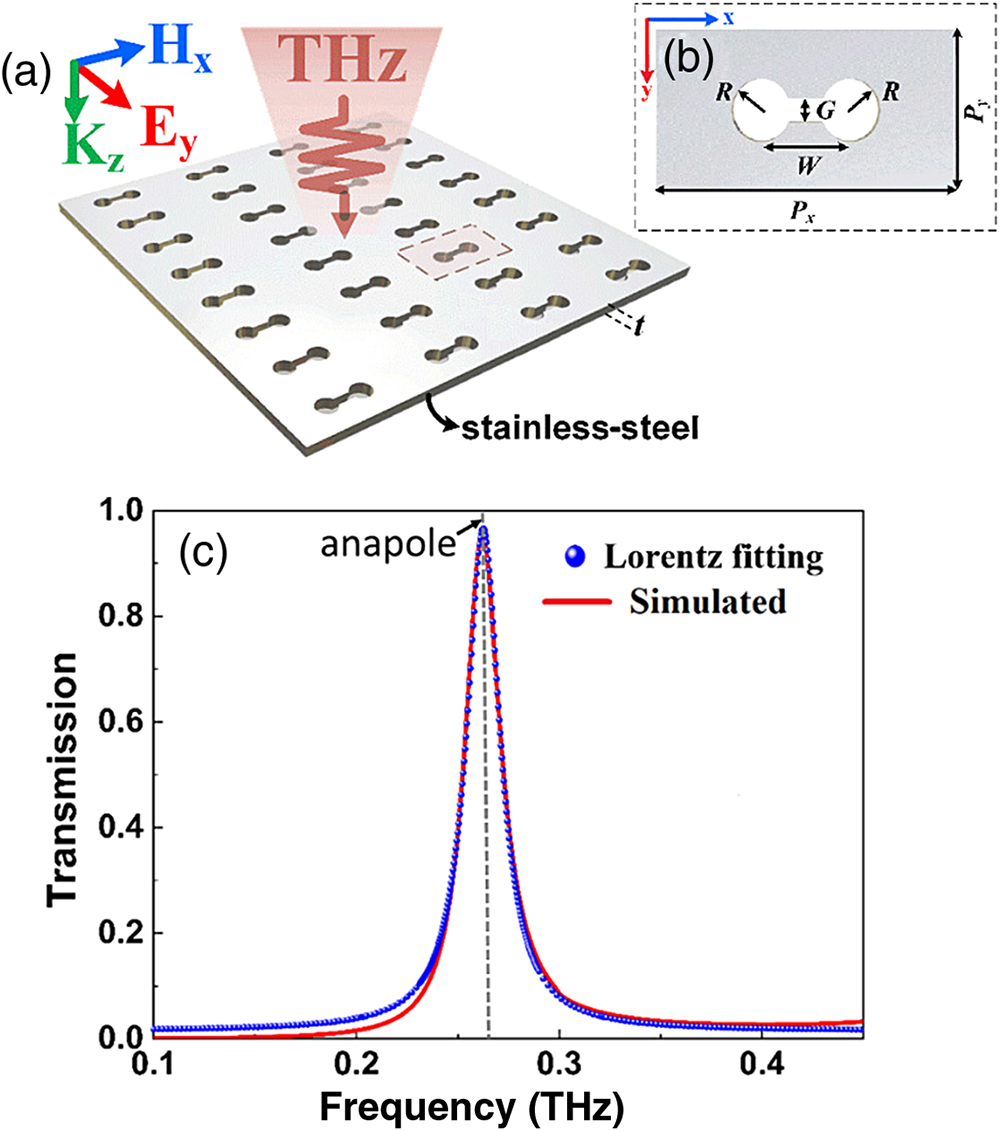
Fig. 1. (a), (b) Schematic of a planar anapole metamaterial with dumbbell-shaped apertures, which is illuminated by a normally incident plane wave with the electric field along the y axis. The structure parameters are radius R , width G , separation between the two circular cuts W , P x = 1,000 μm , P y = 500 μm , and the thickness of the stainless-steel sheet t = 200 μm . (c) Simulated and Lorentz fitted transmission resonance at 0.26 THz for the planar metamaterial when R = 100 , W = 300 , and G = 75 μm .
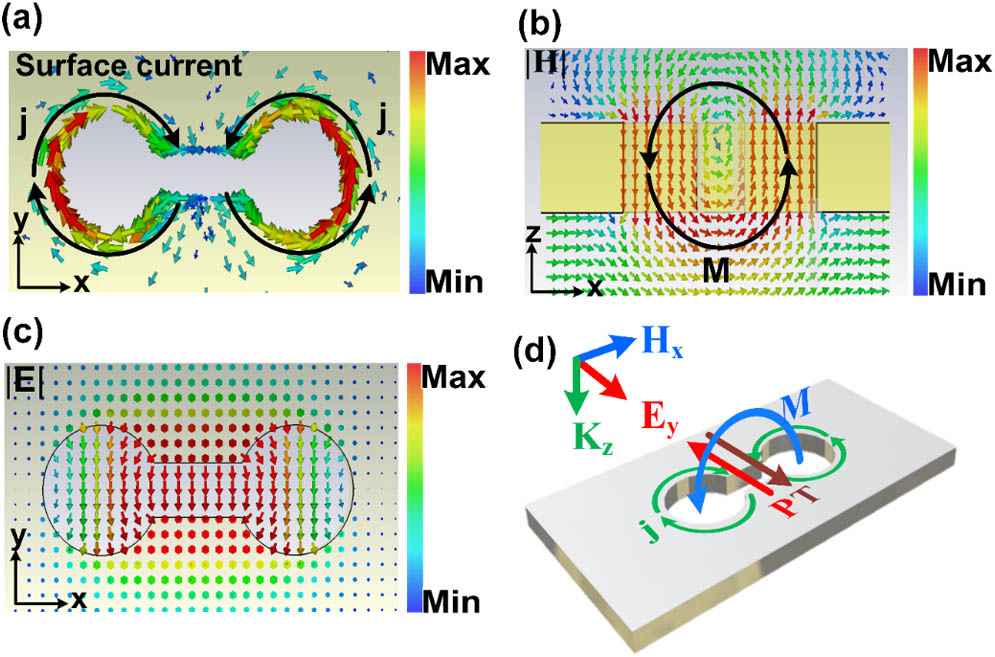
Fig. 2. (a) Surface current in the x -y plane, (b) magnetic field in the x -z plane, and (c) electric field in the x -y plane at the resonance of 0.26 THz for the planar metamaterial. The black arrows represent the direction of the surface current and magnetic field in (a) and (b), respectively. (d) Schematic of the excitation of toroidal dipole T and electric dipole P in the unit cell; M represents the head-to-tail magnetic moment, and j represents the surface current.
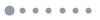
Fig. 3. (a) Five leading scattered powers of multipole decomposition for the planar metamaterial, where P , M , T , QE, and QM are electric dipole, magnetic dipole, toroidal dipole, electric quadrupole, and magnetic quadrupole, respectively. (b) Phase of Cartesian P and i k T . The orange dots represent the phase difference between them. The grey dotted line indicates the location of the corresponding resonant transparency at 0.26 THz.
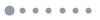
Fig. 4. Transmission spectra of planar metamaterials and corresponding scattered powers of the T and P at resonance (a), (b) with respect to R when W = 300 μm and G = 75 μm ; (c), (d) with respect to W , when R = 100 μm and G = 75 μm ; and (e), (f) with respect to G , when R = 100 μm and W = 300 μm . The phase difference between P and i k T remains constant at π .
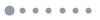
Fig. 5. Three anapole resonance bands produced by varying G when R and W are fixed. In the case of band A: W = 300 μm and R = 100 μm ; band B: W = 150 μm and R = 50 μm ; and band C: W = 100 μm and R = 33 μm .
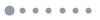
Fig. 6. Measured (solid line) and simulated (dotted line) transmission spectra of four anapole metamaterials with different values of G = 33 , 54, 78, and 95 μm, respectively. Inset: microscopic top view of a fabricated planar anapole metamaterial with R = 100 μm , W = 300 μm , and G = 78 μm .
|
Table 1. Three Anapole Resonance Bands with Different Structural Parameters
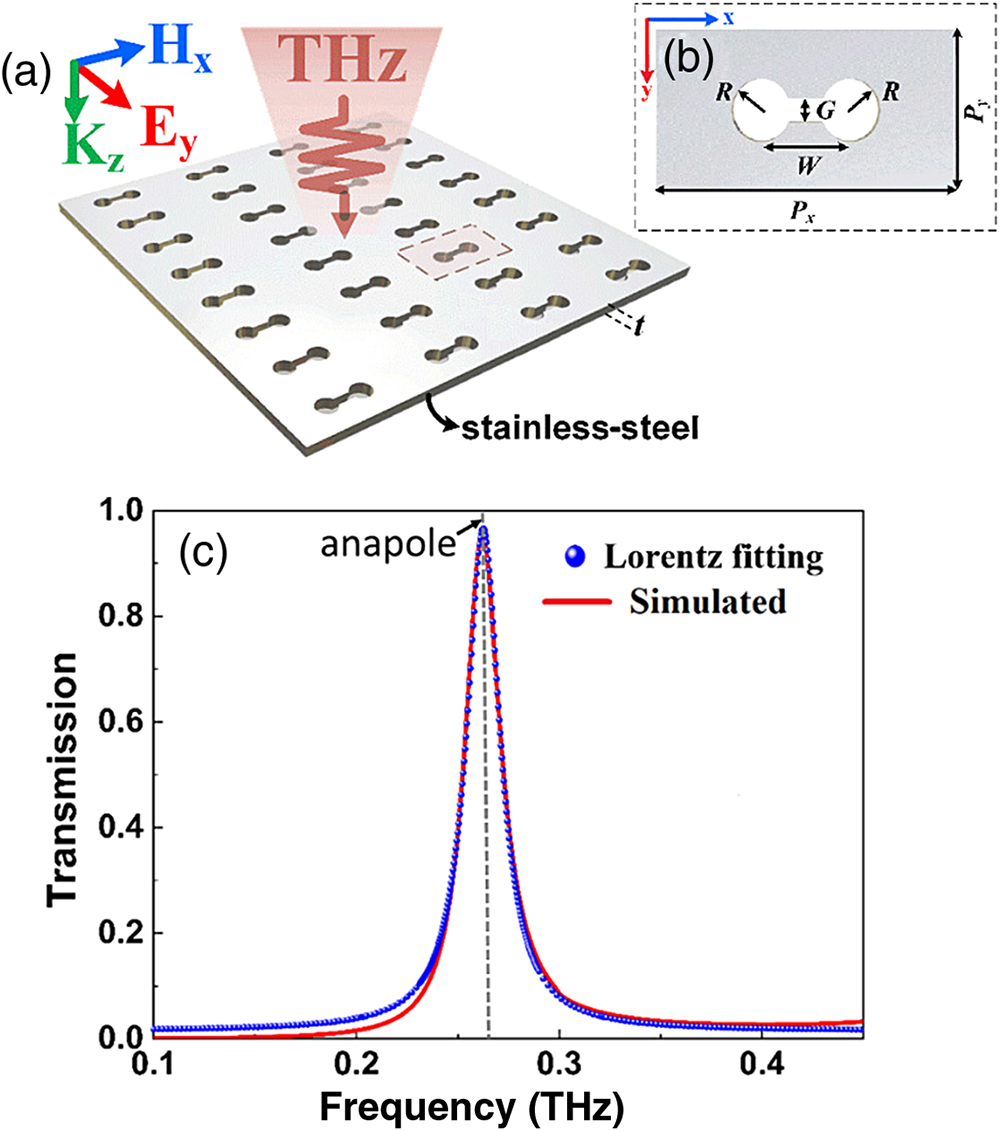
Set citation alerts for the article
Please enter your email address