Abstract
In transition metal dichalcogenides (TMD) flakes, the geometry, such as layer thickness, significantly tune the electronic properties, including bandgap, electron affinity and Fermi level. Such characteristic offers a high degree of freedom to tune the functionality of semiconductor device, once the volatile electronic properties are precisely determined. However, to date, there are still significant uncertainties in determining the Fermi-level alignment of TMD homo- or hetero- junctions, which might lead to significant deviations of band-bending and thus device performance. Here, we utilize the Scanning Kelvin Probe Microscopy (SKPM) to characterize the surface-potential/Fermi-level alignment of TMD homo- or hetero- junctions. Through this effort, a distinct phenomenon is verified where the Fermi-levels of MoS2 and MoTe2 shift towards the intrinsic level with an increasing layer thickness (in other words, the background doping concentration is continuously lowering). Moreover, we show the significant impact of surface contamination (molecular scale) on the surface potential of monolayer TMD. Finally, we fabricate a MoTe2/MoS2 heterojunction, in which we observe the wide depletion region and large photoresponse. Together, those findings might offer a reference to precisely stack van der Waals (vdW) layers as designed for both electronic and optoelectronic applications.Introduction
Transition metal dichalcogenides (TMD),a branch of two-dimensional (2D) layered materials,have attracted continuous attention from both academic and industrial societies due to their intriguing electronic and optoelectronic properties[1-4]. Generally,the bandgap,electron affinity and Fermi-level of layered TMD are tunable with a decreasing layer-thickness[5-9]. Moreover,each monolayer (ML) is connected by van der Waals force[10-12]. It spares TMD materials from surface states/charges and lattice mismatch,thus enabling a freewill stacking of heterostructures[13-14]. Both of these advantages lead to an extremely rich degree of freedom in designing and fabricating nanoscale electronic and optoelectronic devices. However,everything has two sides,the large-scale freedom also means enormous uncertainty in realistic device applications. In detail,the electronic characteristics of TMD materials,like the layer thickness dependent Fermi-level and band-bending,are still out of common sense[6,15]. For this reason,the fabricated homo- or hetero- structures usually deviate from the designed configuration. Note that the theoretical calculations can give a series of reference data[9,16-21]. However,as is well-documented,those results might deviate from the realistic situation[5,7,22],to a certain extent.
Scanning Kelvin Probe Microscopy(SKPM) has become a powerful tool for characterizing electronic properties in TMD,which are crucial for the design and optimization of TMD-based electronic and optoelectronic devices. Li et al. [23] and Feng et al. [24] characterized the surface potential variation of MoS2 using SKPM under different substrates,light intensities,and humidity conditions. Robinson et al. [25] compared the surface potential of MoS2 flakes exfoliated on substrates and suspended flakes using SKPM. Additionally,Wang et al. [26] utilized SKPM to reveal the work function change/Fermi level shift of WSe2 as a function of gate voltage. Furthermore,Zhang et al. [27] confirmed the type-II energy band structure and interlayer charge separation in a MoTe2/MoS2 vdW heterostructure using SKPM. Overall,SKPM provides a non-destructive and high-resolution method for characterizing TMD materials,which could facilitate the development of TMD-based electronic and optoelectronic devices.
In this work,SKPM method is utilized to characterize the surface potential of TMD materials,MoS2 and MoTe2. As a result,a distinct phenomenon has been observed,in which the Fermi-level (EF) of MoS2 and MoTe2 shifts toward the intrinsic level (Ei) with an increasing layer thickness. It indicates that the background doping concentrations of TMD materials are decreasing continuously. These characteristics should be associated with the band structure reconstruction process,in which the formation energy of the intrinsic dopants changes obviously. Finally,we show the alignment of Fermi-level in a typical MoS2/MoTe2 heterostructureand the effect of light illumination on it. The findings disclosed here might provide key information for the design and fabrication of van der Waals devices.
1 Experiments
Homogeneous structures of MoTe2 and MoS2 flakes were mechanically exfoliated onto a silicon substrate covered with a 280 nm-thick thermal oxide layer. To obtain MoTe2/MoS2 heterostructures,MoS2 monolayers were first deposited on Si/SiO2 substrate by chemical vapor deposition (CVD) and a single layer MoTe2 was subsequently transferred onto a selected monolayer of MoS2. All samples were tested in a nitrogen atmosphere to reduce the influence of water and adsorbates,in order to characterize the intrinsic electrical properties of the materials.
A Bruker icon scanning probe microscope with Nanoscope IV controller is used in the experiment. Probes with n-doped Si were selected for both topography and surface potential measurement. We identified thin layers of MoS2 and MoTe2 using an optical microscope,and then used atomic force microscopy (AFM) to characterize the film thickness. The amplitude modulation mode(AM-mode) SKPM was performed to investigate the band alignment of homo- and hetero- structures formed by MoTe2 and MoS2. To explore the interlayer photoexciting effect on MoTe2/MoS2 type-II heterostructures,we performed SKPM analysis under dark and illuminated conditions,with a laser wavelength of 680 nm.
2 Results and discussions
Figures 1(a) and 1(c) show the AFM image and height profile of MoS2 flake that was mechanically exfoliated and transferred onto a Si/SiO2 substrate. The stepped topography helps to identify five distinct layer-thicknesses,namely 1L,2L,3L,4L,and 6L MoS2. The corresponding SKPM image and potential profile are shown in Figs. 1(b) and (d). One can find that each increase in layer thickness (monolayer’s level) would lead to a decrease of surface potential,from 2.2 meV @1L to -8.2 meV @2L,-18 meV @4L and finally -20.3 meV @6L.
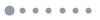
Figure 1.The SKPM experimental results of few-layer MoS2 (a) the AFM image of stepped MoS2,including 1L,2L,3L ,4L and 6L,(b) the SKPM image of the corresponding topography of MoS2,(c) the MoS2 height profile corresponding to the white dashed line marked in (a),(d) the surface potential distribution of few-layer MoS2 extracted from (b),(e) the bandgap and potential of MoS2 as a function of layer thickness,in black and red,respectively (the bandgap data are from the theoretical calculation results in Ref. 5),the potential data at 6L are regional average,(f) evolution of the Fermi level of MoS2 with different layers
It was once thought that oxygen and moisture in the environment would change the surface potential[6],thus responsible for the variable SKPM-signal with an increasing layer thickness. However,in our experiments,all samples were tested in a nitrogen atmosphere to minimize the influence of adsorbates (such as oxygen and water molecules) on the intrinsic electrical properties of TMD. Furthermore,as shown in Fig. 2(c),we observe an opposite trend of the evolution of surface potential in MoTe2 flake,which cannot be explained by the above-mentioned reasons (which predicts a monotonic relationship between potential and layer thickness that is independent of the material category).
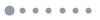
Figure 2.The SKPM experimental results of few-layer MoTe2 (a) the AFM image of stepped MoTe2,including 1L,2L and 3L,(b) the SKPM image of the corresponding topography of MoTe2,the light blue dashed lines are extensions of the potential boundary,The white triangles in white dashed ovals mark topography and potentials due to the module absorption or contamination,(c) the surface potential distribution and corresponding topography of the few-layer MoTe2 extracted from (a) and (b),(d) the height and potential curves corresponding to the white dotted line in the MoTe2 monolayer region in (b),(e) evolution of the Fermi level of MoTe2 with different layers,(f) the number and average potential of adsorbates with different diameter ranges in the monolayer region of MoTe2
Here,we attribute the phenomenon in Figs. 1(c) and 1(d) to the intrinsic variation of the Fermi level. This is because the band structure of van der Waals materials is reconstructed with an increasing layer thickness. It could strongly tune the background doping property,e. g. by altering the defect forming energy. Figure 1(e) compares the bandgap with the surface potential at different layer thicknesses. One can find that both characters follow the same trend of evolution,which might be the direct evidence to support the judgement.
To further test this,we performed the SKPM experiment on another TMD material,MoTe2. The typical result is shown in Fig. 2. The morphology image (Fig. 2(a)) helps to identify three distinct regions,1L,2L and 3L MoTe2. Correspondingly,the surface potential exhibits an obvious enhancement in the thick-layer counterpart of MoTe2 (Fig. 2(b)). It is contrary to that of MoS2,where the potential increases with an increasing layer thickness. Figures 1(f) and 2(e) schematically show the band structures of MoS2 and MoTe2,respectively. The Fermi-level is carefully labelled to show the opposite evolution processes.
It is worth noting that there are space charge regions (SCR) in the MoTe2 sample,especially at the 1L/2L and 2L/3L interfaces (Fig. 2(b),marked by white triangles and blue dashed lines). It arises from the band offset between different layer-thickness regions,which can be defined as the depletion region of the layer junction. Such a characteristic is much more obvious in MoTe2,but almost invisible in MoS2. The difference in charge carrier concentration might be responsible for the phenomenon. In detail,MoTe2 is lightly p-doped (a well-known bipolar material) [28-30],MoS2 is heavily n-doped[31-32]. Thus,the depletion region of MoTe2 could be ordered wider than that of MoS2.
Another interesting phenomenon is that the fluctuations of surface potential are clearly recorded in our SKPM experiments (Fig. 2(b)),even if they are sub-nanometer scale and originate from molecules absorption or contamination. As shown in Figs. 2(b) and 2(d),in the monolayer MoTe2 region,there is a high density of potential fluctuation (as high as 20 meV),which arises from the sub-nanometer molecules that are incorporated during the mechanical exfoliation process. Figure 2(f) shows a statistical analysis on the diameter and potential of the adsorbed molecules or molecule clusters in the monolayer MoTe2. One can find that the diameter of molecular clusters spans from sub-nanometer to 10 nanometers; the most frequently found molecules are sub-2 nm ones,which account for 77% of the whole community. The histogram plot shows the relation between the potential fluctuations on the scale of absorbed molecule. It makes sense that the potential fluctuation rises from 11 meV to 27 meV with an increase of the molecule diameter.
Furthermore,we prepared a 1L MoTe2-1L MoS2 heterostructure. As depicted in Fig. 3(a),the sample was fabricated by transferring a monolayer MoTe2 onto the 1L-MoS2. In SKPM experiments,the MoTe2 film shows a 104 mV drop in potential. It indicates that the 1L MoTe2-1L MoS2 heterostructure is a typical pn junction. At the same time,the SKPM experiment helps to identify the depletion region of such vdW hetero-junction. As shown in Fig. 3(c),the transition region between n-MoS2 and p-MoTe2 is a classic space charge region. Its width is as large as 750 nm. A more detailed analysis confirms this conclusion. Figure 3(d) plots the dependence of potential drop on the width of MoTe2 strip. One can find that the potential drop increases significantly (58 to 109 mV) as the MoTe2 width increases from 350 to 750 nm. In this specific region,the MoTe2 layer is fully depleted,thus the potential drop is highly dependent on the layer width. By contrast,when the MoTe2 width is over 750 nm,the potential drop is constant. It implies that the junction cannot deplete more material. The 750 nm should be the final width of the depletion region. It is also worth noting that such vdW hetero-junction shows a significant response to light illumination[27],since the potential drop increases by ~20 mV.
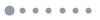
Figure 3.The SKPM experimental results of MoTe2/MoS2 vdW heterostructures (a) the AFM 3D image of MoTe2/MoS2 vdW heterostructure,(b) the SKPM 3D image of corresponding topography,(c) the topography and surface potential distributions corresponding to the white dashed line in (b),(d) the size dependence of the potential difference between the MoTe2/MoS2 vdW heterojunction and MoS2 under dark and light conditions,the inset shows the changes in surface potential of the MoTe2/MoS2 heterostructure under dark and light conditions
3 Conclusions
We explore the effect of layer thickness on the band alignment of homo- and hetero-structures formed by few-layer MoS2 and MoTe2. As the layer thickness increases,the Fermi level of MoS2 decreases while the Fermi level of MoTe2 increases,resulting in opposite evolution trends. The observed space charge region in MoTe2 is attributed to differences in carrier concentrations,while significant surface potential fluctuations in the monolayer region reveal the effects of impurity adsorbents and non-ideal storage conditions. Our findings offer guidance for the fabrication of 2D material-based devices. We also prepared a vdW heterostructure of monolayers MoS2 and MoTe2,and observed a classical space charge region with a final depletion region width of 750 nm. The potential drop of the vdW heterostructure increased by 20 mV under illumination,indicating a good photo-response.
In summary,this work expands our understanding of the electronic structure of layered TMD materials,demonstrating that band alignment can be modified by adjusting the layer thickness. This property likely extends to other TMD materials,offering important guidance for the design of next-generation electronic and optoelectronic devices based on TMD homo- or hetero-structures.
References
[1] C L Tan, Z C Lai, H Zhang. Ultrathin Two-Dimensional Multinary Layered Metal Chalcogenide Nanomaterials. Advanced Materials, 29, 1701392(2017).
[2] C Xie, C Mak, X M Tao et al. Photodetectors Based on Two-Dimensional Layered Materials Beyond Graphene. Advanced Functional Materials, 27, 1603886(2017).
[3] G R Bhimanapati, Z Lin, V Meunier et al. Recent Advances in Two-Dimensional Materials beyond Graphene. Acs Nano, 9, 11509-11539(2015).
[4] X Z Bao, Q D Ou, Z Q Xu et al. Band Structure Engineering in 2D Materials for Optoelectronic Applications. Advanced Materials Technologies, 3, 1800072(2018).
[5] K F Mak, C Lee, J Hone et al. Atomically Thin MoS2: A New Direct-Gap Semiconductor. Physical Review Letters, 105, 136805(2010).
[6] Y Li, C Y Xu, L Zhen. Surface potential and interlayer screening effects of few-layer MoS2 nanoflakes. Applied Physics Letters, 102, 143110(2013).
[7] C Ruppert, O B Aslan, T F Heinz. Optical Properties and Band Gap of Single- and Few-Layer MoTe2 Crystals. Nano Letters, 14, 6231-6236(2014).
[8] H G Kim, H J Choi. Thickness dependence of work function, ionization energy, and electron affinity of Mo and W dichalcogenides from DFT and GW calculations. Physical Review B, 103, 085404(2021).
[9] H Lee, S Deshmukh, J Wen et al. Layer-Dependent Interfacial Transport and Optoelectrical Properties of MoS2 on Ultraflat Metals. Acs Applied Materials & Interfaces, 11, 31543-31550(2019).
[10] A K Geim, I V Grigorieva. Van der Waals heterostructures. Nature, 499, 419-425(2013).
[11] K S Novoselov, A Mishchenko, A Carvalho et al. 2D materials and van der Waals heterostructures. Science, 353, aac9439(2016).
[12] S Das, H Y Chen, A V Penumatcha et al. High Performance Multilayer MoS2 Transistors with Scandium Contacts. Nano Letters, 13, 100-105(2013).
[13] M Y Li, C H Chen, Y M Shi et al. Heterostructures based on two-dimensional layered materials and their potential applications. Materials Today, 19, 322-335(2016).
[14] W H Zheng, B Y Zheng, C L Yan et al. Direct Vapor Growth of 2D Vertical Heterostructures with Tunable Band Alignments and Interfacial Charge Transfer Behaviors. Advanced Science, 6, 1802204(2019).
[15] H Xia, M Luo, W J Wang et al. Pristine PN junction toward atomic layer devices. Light-Science & Applications, 11, 170(2022).
[16] T Cheiwchanchamnangij, W R L Lambrecht. Quasiparticle band structure calculation of monolayer, bilayer, and bulk MoS2. Physical Review B, 85, 205302(2012).
[17] T Boker, R Severin, A Muller et al. Band structure of MoS2, MoSe2, and alpha-MoTe2: Angle-resolved photoelectron spectroscopy and ab initio calculations. Physical Review B, 64, 235305(2001).
[18] J Kang, S Tongay, J Zhou et al. Band offsets and heterostructures of two-dimensional semiconductors. Applied Physics Letters, 102, 012111(2013).
[19] W D Hu, Z H Ye, L Liao et al. 128 x 128 long-wavelength/mid-wavelength two-color HgCdTe infrared focal plane array detector with ultralow spectral cross talk. Optics Letters, 39, 5130-5133(2014).
[20] C Gong, H J Zhang, W H Wang et al. Band alignment of two-dimensional transition metal dichalcogenides: Application in tunnel field effect transistors. Applied Physics Letters, 103, 053513(2013).
[21] W D Hu, X S Chen, Z H Ye et al. A hybrid surface passivation on HgCdTe long wave infrared detector with in-situ CdTe deposition and high-density Hydrogen plasma modification. Applied Physics Letters, 99, 091101(2011).
[22] S Mcdonnell, A Azcatl, R Addou et al. Hole Contacts on Transition Metal Dichalcogenides: Interface Chemistry and Band Alignments. Acs Nano, 8, 6265-6272(2014).
[23] F Li, J J Qi, M X Xu et al. Layer Dependence and Light Tuning Surface Potential of 2D MoS2 on Various Substrates. Small, 13, 1603103(2017).
[24] Y L Feng, K L Zhang, H Li et al. In situ visualization and detection of surface potential variation of mono and multilayer MoS2 under different humidities using Kelvin probe force microscopy. Nanotechnology, 28, 295705(2017).
[25] B J Robinson, C E Giusca, Y T Gonzalez et al. Structural, optical and electrostatic properties of single and few-layers MoS2: effect of substrate. 2d Materials, 2, 015005(2015).
[26] Z G Wang, Q Li, Y F Chen et al. The ambipolar transport behavior of WSe2 transistors and its analogue circuits. Npg Asia Materials, 10, 703-712(2018).
[27] K N Zhang, T N Zhang, G H Cheng et al. Interlayer Transition and Infrared Photodetection in Atomically Thin Type-II MoTe2/MoS2 van der Waals Heterostructures. Acs Nano, 10, 3852-3858(2016).
[28] A Pezeshki, S Hossein, H Shokouh et al. Electric and Photovoltaic Behavior of a Few-Layer alpha-MoTe2/MoS2 Dichalcogenide Heterojunction. Advanced Materials, 28, 3216-3222(2016).
[29] Y F Lin, Y Xu, S T Wang et al. Ambipolar MoTe2 Transistors and Their Applications in Logic Circuits. Advanced Materials, 26, 3263-3269(2014).
[30] S Fathipour, N Ma, W S Hwang et al. Exfoliated multilayer MoTe2 field-effect transistors. Applied Physics Letters, 105, 192101(2014).
[31] B Radisavljevic, A Radenovic, J Brivio et al. Single-layer MoS2 transistors. Nature Nanotechnology, 6, 147-150(2011).
[32] H Q Liu, K Ba, S F Gou et al. Reversing the Polarity of MoS2 with PTFE. Acs Applied Materials & Interfaces, 13, 46117-46124(2021).