
- Chinese Optics Letters
- Vol. 19, Issue 11, 112201 (2021)
Abstract
Keywords
1. Introduction
In recent years, photovoltaic agricultural technology has developed rapidly[
In this Letter, we propose a promising solution based on spectral separation to select the red, blue, and far-red light from sunlight for photosynthesis of plants, and the rest of the sunlight is used to concentrate on photovoltaic panels to generate electricity. The key of the solution is to design a multi-passband filter film at low cost. The multiplication co-extrusion (MCE) process, which is capable of economically and continuously producing, has been adopted to prepare alternately superimposed multilayer film[
2. Multilayer Stacks Design Method
The film design of polymer multilayer periodic structures should firstly consider the choice of polymer material combination. The refractive indexes of optical polymer materials are generally between 1.4 and 1.6. The greater the difference in refractive index, the easier it is to design a multilayer optical film with higher reflectivity, wider reflection bandwidth, and fewer layers. The polymethyl methacrylate (PMMA, refractive index
Sign up for Chinese Optics Letters TOC. Get the latest issue of Chinese Optics Letters delivered right to you!Sign up now
For better understanding the effects of microstructure on the optical performance, we use a modified transfer matrix theory to calculate the reflectivity of a non-absorbing multilayer system, taking into account multiple reflections. In the following, we sketch the essential steps of the method in Refs. [10–12].
The reflectance of the multilayer film is given as
The MCE process usually adopts equal division and multiplication to obtain a film structure of equal thickness period. The spectral characteristics of the Bragg reflective film with a periodic structure of equal thickness only have a reflection band with a single central wavelength, and the reflection bandwidth is limited. As shown in Fig. 1(a), using PC and PMMA as the material combination, and 410 nm as the reference wavelength, the simulation by TFCalc shows that the reflectivity of the 128-layer multilayer film is almost close to 100%. Due to the small difference in refractive index between the two materials, the reflection bandwidth is only about 20 nm.
Figure 1.Broadening of reflection bandwidth: (a) reflectivity of different layers of PC/PMMA multilayer film; (b) reflectance spectra of periodic films with different thicknesses.
In order to realize the design of complex multi-passband optical film system, it is necessary to obtain a series of high-reflection films with different passband positions and wider reflection bandwidth.
Figure 1(b) shows the method of broadening the reflection bandwidth. Take 64[HL] (H = PC, L = PMMA) as the basic film stack1 with a film stack of equal thickness period (410 nm as the reference wavelength). Stack2, which is 1.04 times thicker than stack1, has a center wavelength of 430 nm and the same bandwidth as stack1. When stack2 and stack1 are superimposed together, the bandwidth is doubled. Using more film stacks can achieve an unequal thickness periodic structure with stack thickness gradient.
Polymer film can be stretched and thinned under the glass transition temperature, so the different passband positions and the width of the reflection bandwidth can be adjusted arbitrarily. As shown in Fig. 2, by superimposing stacks with different passband positions, when the short cut-off and long cut-off of each high-reflection band avoid reflections in the 450 nm, 660 nm, and 730 nm bands, a three-passband polymer multilayer optical film can be obtained. As shown in Fig. 3, this film design can accurately and selectively transmit the red, blue, and far-red light in the sunlight, which can meet the light quality conditions required by plant photosynthesis.
Figure 2.Superimposing of film stacks at different passband positions.
Figure 3.Three-passband multilayer film suitable for plant photosynthesis.
3. Results and Discussion
Low-cost polymer multilayer optical films are economically feasible for high-value-added photovoltaic agricultural systems. Limited by the experimental conditions, in this Letter, we select two single-passband polymer multilayer optical film products (Filter A and Filter B) produced by 3M company with different bandpass positions for splicing to obtain a double-passband polymer multilayer optical film (Filter C), which are shown in Fig. 4. This transmission spectrum of Filter C satisfies all of the light bands required for plant growth. Obviously, it can be seen that the transmission bandwidth of Filter C covers 545–792 nm, which is relatively “surplus” for the red and far-red light required for plant photosynthesis. From the perspective of comprehensive utilization of solar energy, the photovoltaic agricultural system only needs to accurately transmit 450 nm, 660 nm, and 730 nm, and the rest of the bands of light can be used to collect and generate more electricity. Our team has built a 640-layer polymer multilayer optical film co-extrusion casting experiment platform and unidirectional stretching device. Through process design and optimization, it is expected to obtain a spectroscopic film that is more suitable for plant photosynthesis.
Figure 4.Spectrum of the double-passband polymer multilayer optical film.
The demonstration system of the photovoltaic agricultural system was constructed using a double-passband polymer optical film. Since the incident light angle of sunlight changes every day, the incident light angle will have a blue shift effect on the spectral characteristics of the multilayer optical film. When the incident angle is 40°, the center wavelength position of the reflection band blue shifts by about 50 nm. Therefore, in this application scenario, the trough-type low-power concentrator system needs to adopt a dual-axis sun tracking system to maintain the vertical incidence of sunlight.
As shown in Fig. 5, since the relative spectral response of the crystalline silicon solar cell in the short-wavelength band of blue and red light is low, the blue and red light are separated and transmitted for the photosynthesis of plants, which has little effect on photovoltaic power generation efficiency. Sonneveld et al.[
Figure 5.Multi-passband filter film applied to the photovoltaic agricultural system: (a) solar radiation and spectral response of crystalline silicon cells; (b) biaxial tracking photovoltaic agricultural system; (c) spectra required for plant photosynthesis.
Polymer materials generally have poor UV resistance and have a short service life when used outdoors for a long time. The UV band can be reflected by adjusting the thickness of the film period. In addition to the first-order reflection, a polymer multilayer film can exhibit higher-order reflections. The wavelengths at which reflections of the various orders appear are given by[
4. Conclusion
A 450 nm, 660 nm, and 730 nm three-passband filter was realized by superimposing stacks of different band positions. Using the design method in this Letter, the selective transmission spectrum of the polymer multilayer optical film can be arbitrarily adjusted. The feasibility of photovoltaic agriculture was confirmed by the power generation efficiency and actual plant growth. The polymer filter has an obvious low-cost advantage; not only can it be used in photovoltaic agricultural systems to efficiently use solar energy, but it can also be used in fields such as heat insulation, anti-UV, and infrared shielding.
References
[1] A. Weselek, A. Ehmann, S. Zikeli, I. Lewandowski, P. Högy. Agrophotovoltaic systems: applications, challenges, and opportunities: a review. Agron. Sustain. Dev., 39, 35(2019).
[2] R. Aroca-Delgado, J. Pérez-Alonso, Á. J. Callejón-Ferre, B. Velázquez-Martí. Compatibility between crops and solar panels: an overview from shading systems. Sustainability, 10, 743(2018).
[3] C. Dupraz, H. Marrou, G. Talbot, L. Dufour, A. Nogier, Y. Ferard. Combining solar photovoltaic panels and food crops for optimising land use: towards new agrivoltaic schemes. Renewable Energy, 36, 2725(2011).
[4] A. Marucci, D. Monarca, M. Cecchini, A. Colantoni, A. Manzo, A. Cappuccini. The semitransparent photovoltaic films for Mediterranean greenhouse: a new sustainable technology. Math. Prob. Eng., 2012, 451934(2012).
[5] M. Kasahara, T. Kagawa, Y. Sato, T. Kiyosue, M. Wada. Phototropins mediate blue and red light-induced chloroplast movements in Physcomitrella patens. Plant Physiol., 135, 1388(2004).
[6] H. Hwang, S. An, B. Lee, C. Chun. Improvement of growth and morphology of vegetable seedlings with supplemental far-red enriched LED lights in a plant factory. Horticulturae, 6, 109(2020).
[7] J. H. Andrews, M. Crescimanno, N. J. Dawson, G. Mao, J. B. Petrus, K. D. Singer, E. Baer, H. Song. Folding flexible co-extruded all-polymer multilayer distributed feedback films to control lasing. Opt. Express, 20, 15580(2012).
[8] M. F. Weber, C. A. Stover, L. R. Gilbert, T. J. Nevitt, A. J. Ouderkirk. Giant birefringent optics in multilayer polymer mirrors. Science, 287, 2451(2000).
[9] K. D. Singer, T. Kazmierczak, J. Lott, H. Song, Y. Wu, J. Andrews, E. Baer, A. Hiltner, C. Weder. Melt-processed all-polymer distributed Bragg reflector laser. Opt. Express, 16, 10358(2008).
[10] T. Alfrey, E. F. Gurnee, W. J. Schrenk. Physical optics of iridescent multilayered plastic films. Polym. Eng. Sci., 9, 400(1969).
[11] H. Xiong, Y. Tang, L. Hu, H. Li. An ORMOSIL porous double-layer broadband antireflective coating. Chin. Opt. Lett., 17, 033101(2019).
[12] J. Tang, J. Xu, Z. Zheng, H. Dong, J. Dong, S. Qian, J. Guo, L. Jiang, Y. Xiang. Graphene Tamm plasmon-induced giant Goos–Hänchen shift at terahertz frequencies. Chin. Opt. Lett., 17, 020007(2019).
[13] P. J. Sonneveld, G. L. A. M. Swinkels, G. P. A. Bot, G. Flamand. Feasibility study for combining cooling and high grade energy production in a solar greenhouse. Biosyst. Eng., 105, 51(2010).
[14] P. J. Sonneveld, G. L. A. M. Swinkels, J. Campen, B. A. J. van Tuijl, H. J. J. Janssen, G. P. A. Bot. Performance results of a solar greenhouse combining electrical and thermal energy production. Biosyst. Eng., 106, 48(2010).
[15] J. A. Radford, T. Alfrey, W. J. Schrenk. Reflectivity of iridescent coextruded multilayered plastic films. Polym. Eng. Sci., 13, 216(1973).
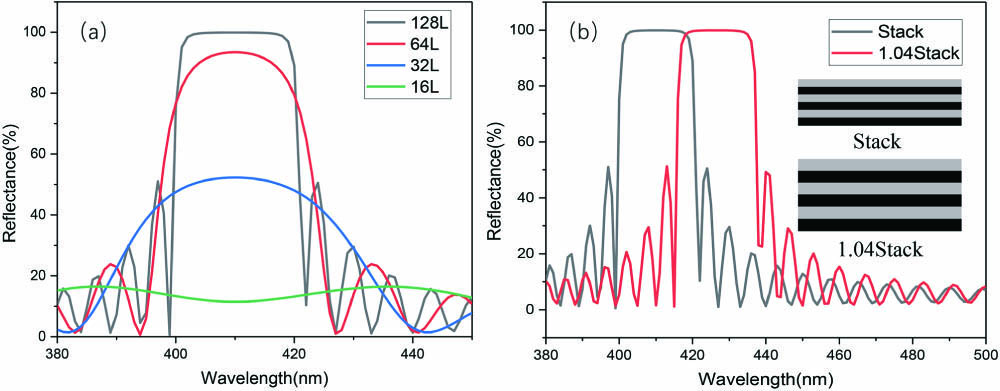
Set citation alerts for the article
Please enter your email address