Sb-doped

- Chinese Optics Letters
- Vol. 21, Issue 4, 041605 (2023)
Abstract
1. Introduction
In recent years, the
It has been reported that the element Sb is often used as an effective dopant to improve the conductivity of semiconductor crystals[27,28]. The
Sign up for Chinese Optics Letters TOC. Get the latest issue of Chinese Optics Letters delivered right to you!Sign up now
2. Experimental Procedure
Un-doped and Sb-doped
The crystal structures were determined by X-ray diffraction (XRD) with a Rigaku D/max 2550. The X-ray rocking curve of the Sb-doped
3. Results and Discussion
Figure 1 shows the photos of the as grown Sb-doped
Figure 1.Photos of the as grown Sb-doped β-Ga2O3 crystals. (a) 0.1% Sb; (b) 1.0% Sb; (c) 2.0% Sb.
Figure 2 shows the XRD patterns of the database (JCPDF: 41-1103) and Sb-doped
Figure 2.XRD patterns of the database (PDF: 41-1103) and Sb-doped β-Ga2O3 single crystals.
Figure 3.X-ray rocking curve for the (400) plane of 1.0% Sb-doped β-Ga2O3 single crystal.
The actual concentration of Sb in the Sb-doped crystals and in the polycrystalline rods, tested using ICP-AES, is listed in Table 1. Evidently, the concentration of Sb in the crystals and in the polycrystalline rods was much lower than that in the raw materials.
Sample 1 | Sample 2 | Sample 3 | |
---|---|---|---|
Nominal composition Sb (%) | 0.1 | 1.0 | 2.0 |
Rod [ICP-AES (%)] | 0.0142 | 0.0206 | 0.0309 |
Crystal [ICP-AES (%)] | 0.0101 | 0.0157 | 0.0237 |
Effective segregation coefficient | 0.71 | 0.76 | 0.77 |
Table 1. ICP-AES Data of Sb-Doped
Room temperature Raman spectra of Sb-doped
Figure 4.Room temperature Raman spectra of Sb-doped β-Ga2O3 single crystals.
Figure 5.Room temperature Raman spectra of Sb-doped β-Ga2O3 single crystals in the 300–460 cm−1 wavenumber range.
Room temperature electrical properties of the un-doped and Sb-doped
Samples | Carrier Type | Carrier Concentration (cm−3) | Mobility (cm2 · V−1 · s−1) | Resistivity (Ω · cm) |
---|---|---|---|---|
n | 153.1 | 0.603 | ||
n | 134.7 | 0.064 | ||
n | 126.4 | 0.037 | ||
n | 108.7 | 0.017 |
Table 2. Room-Temperature Hall Data of the Un-Doped and Sb-Doped
Figure 6 shows the room temperature optical transmittance spectra of Sb-doped
Figure 6.Optical transmittance of un-doped and Sb-doped β-Ga2O3 crystals. (a) 200–2000 nm (including their carrier concentrations), (b) 250–300 nm.
4. Conclusion
In summary, Sb-doped
References
[1] J. Blevins, G. Yang. On optical properties and scintillation performance of emerging Ga2O3 crystal growth, emission mechanisms and doping strategies. Mater. Res. Bull., 144, 111494(2021).
[2] J. Zhang, J. Shi, D. Qi, L. Chen, K. H. L. Zhang. Recent progress on the electronic structure, defect, and doping properties of Ga2O3. APL Mater., 8, 020906(2020).
[3] L. Jiang, J. Liu, L. Hu, L. Zhang, A. Tian, W. Xiong, X. Ren, S. Huang, W. Zhou, M. Ikeda, H. Yang. Reduced threshold current density of GaN-based green laser diode by applying polarization doping p-cladding layer. Chin. Opt. Lett., 19, 121404(2021).
[4] M. Higashiwaki, K. Sasaki, A. Kuramata, T. Masui, S. Yamakishi. Gallium oxide (Ga2O3) metal-semiconductor field-effect transistors on single-crystal β-Ga2O3 (010) substrates. Appl. Phys. Lett., 100, 013504(2012).
[5] W. Zhou, C. Xia, Q. Sai, H. Zhang. Controlling n-type conductivity of β-Ga2O3 by Nb doping. Appl. Phys. Lett., 111, 242103(2017).
[6] T. Oshima, T. Okuno, S. Fujiata. Ga2O3 thin film growth on c-plane sapphire substrates by molecular beam epitaxy for deep-ultraviolet photodetectors. Jpn. Appl. Phys., 46, 11(2007).
[7] D. Madadi, A. A. Orouji. Scattering mechanisms in β-Ga2O3 junctionless SOI MOSFET: investigation of electron mobility and short channel effects. Mater. Today. Commun., 26, 102044(2021).
[8] F. Zhou, H. Gong, W. Xu, X. Yu, Y. Xu, H. Lu. 1.95-kV beveled-mesa NiO/β-Ga2O3 heterojunction diode with 98.5% conversion efficiency and over million-times overvoltage ruggedness. IEEE Trans. Electron Devices, 37, 1223(2022).
[9] D. Dhanabalan, V. Ananthu, K. V. Akshita, S. Bhattacharya, E. Varadarajan, S. Ganesamoorthy, S. M. Babu, V. Natarajan, S. Verma, M. Srivatsava, S. Lourdudoss. Studies on Schottky barrier diodes fabricated using single-crystal wafers of β-Ga2O3 grown by the optical floating zone technique. Phys. Status Solidi B, 259, 2100496(2022).
[10] J. Kim, J. Kim. Monolithically integrated enhancement-mode and depletion-mode β-Ga2O3 MESFETs with graphene-gate architectures and their logic applications. ACS Appl. Mater. Interfaces, 12, 7310(2020).
[11] L. Han, Y. Gao, S. Hang, C. Chu, Y. Zhang, Q. Zheng, Q. Li, Z. Zhang. Impact of p-AlGaN/GaN hole injection layer on GaN-based bertical cavity surface emitting laser diodes [Invited]. Chin. Opt. Lett., 20, 031402(2022).
[12] L. Su, W. Xu, D. Zhou, F. Ren, D. Chen, R. Zhang, Y. Zheng, H. Lu. Avalanche mechanism analysis of 4H-SiC n-i-p and p-i-n avalanche photodiodes working in Geiger mode. Chin. Opt. Lett., 19, 092501(2021).
[13] L. Zhou, C. Wang, A. Yi, C. Shen, Y. Zhu, K. Huang, M. Zhou, J. Zhang, X. Ou. Photonic crystal nanobeam cavities based on 4H-silicon carbide on insulator. Chin. Opt. Lett., 20, 031302(2022).
[14] P. Li, Y. Bu, D. Chen, Q. Sai, H. Qi. Investigation of the crack extending downward along the seed of the β-Ga2O3 crystal grown by the EFG method. CrystEngComm, 23, 6300(2021).
[15] H. Cui, H. F. Mohamed, C. Xia, Q. Sai, W. Zhou, H. Qi, J. Zhao, J. Si, X. Ji. Tuning electrical conductivity of β-Ga2O3 single crystals by Ta doping. J. Alloy. Compd., 788, 925(2019).
[16] J. Jesenovec, C. Remple, J. Huso, B. Dutton, P. Toews, M. D. McCluskey, J. S. McCloy. Photodarkening and dopant segregation in Cu-doped β-Ga2O3 Czochralski single crystals. J. Cryst. Growth., 578, 126419(2022).
[17] E. Ohba, T. Kobayashi, T. Taishi, K. Hoshikawa. Growth of (100) and (001) β-Ga2O3 single crystals by vertical Bridgman method. J. Cryst. Growth., 556, 125990(2021).
[18] Y. Tomm, P. Reiche, D. Klimm, T. Fukuda. Czochralski grown Ga2O3 crystals. J. Cryst. Growth., 220, 510(2000).
[19] M. Yamaga, E. G. Villora, K. Shimamura, N. Ichinose. Donor structure and electric transport mechanism in β-Ga2O3. Phys. Rev. B, 68, 155207(2003).
[20] Y. Li, C. Yang, L. Wu, R. Zhang. Electrical and optical properties of Si-doped Ga2O3. Mod. Phys. Lett. B, 31, 1750172(2017).
[21] B. Fu, G. Jian, W. Mu, Y. Li, H. Wang, Z. Jia, Y. Li, S. Long, Y. Shi, X. Tao. Crystal growth and design of Sn-doped β-Ga2O3: morphology, defect and property studies of cylindrical crystal by EFG. J. Alloy. Compd., 896, 1628630(2022).
[22] E. G. Villora, K. Shimamura, Y. Yoshikawa, T. Ujiie, K. Aoki. Electrical conductivity and carrier concentration control in β-Ga2O3 by Si doping. Appl. Phys. Lett., 92, 202120(2008).
[23] Q. Wu, H. Tang, L. Su, P. Luo, X. Qian, F. Wu, J. Xu. Study on growth and properties of Ge:β-Ga2O3 single crystal by optical floating zone method. J. Synth. Cryst., 45, 6(2016).
[24] Z. Galazka, K. Irmscher, R. Schewski, I. M. Hanke, M. Pietsch, S. Ganschow, D. Klimm, A. Dittmar, A. Fiedler, T. Schroeder, M. Bickermann. Czochralski-grown bulk β-Ga2O3 single crystal doped with mono-, di-, tri-, and tetravalent ions. J. Cryst. Growth., 529, 125297(2020).
[25] F. Alema, G. Seryogin, A. Osinsky, A. Osinsky. Ge doping of β-Ga2O3 by MOCVD. APL Mater., 9, 091102(2021).
[26] A. Mauze, Y. Zhang, T. Itoh, E. Ahmadi, J. Speck. Sn doping of (010) β-Ga2O3 films grown by plasma-assisted molecular beam epitaxy. Appl. Phys. Lett., 117, 222102(2020).
[27] R. Nasser, J. Song, H. Elhouichet. Epitaxial growth and properties study of p-type doped ZnO:Sb by PLD. Superlattices Microstruct., 155, 106908(2021).
[28] W. Sinornate, H. Mimura, W. Pecharapa. Structural, morphological, optical, and electrical properties of Sol-Gel derived Sb-doped ZnO thin films annealed under different atmospheres. Phys. Status Solidi A, 218, 2000233(2021).
[29] S. F. Mayer, J. E. Rodrigues, C. Marini, M. T. Fernandez-Diaz, H. Falcon, M. C. Asensio, J. A. Alonso. A comprehensive examination of the local-and long-range structure of Sb6O13 pyrochlore oxide. Sci. Rep., 10, 16956(2020).
[30] J. Zhang, C. Xia, Q. Deng, W. Xu, H. Shi, F. Wu, J. Xu. Growth and characterization of new transparent conductive oxides single crystals β-Ga2O3:Sn. J. Phys. Chem. Solids, 67, 1656(2006).
[31] H. Liu, N. Zhang, J. Yin, C. Xia, Z. Feng, K. He, L. Wan, H. F. Mohamed. Characterization of defect levels in β-Ga2O3 single crystals doped with tantalum. CrystEngComm., 23, 2835(2021).
[32] E. G. Villora, M. Yamaga, T. Inoue, S. Yabasi, Y. Masui, T. Sugawara, T. Fukuda. Optical spectroscopy study on β-Ga2O3. Jpn. J. Appl. Phys., 41, 622(2002).
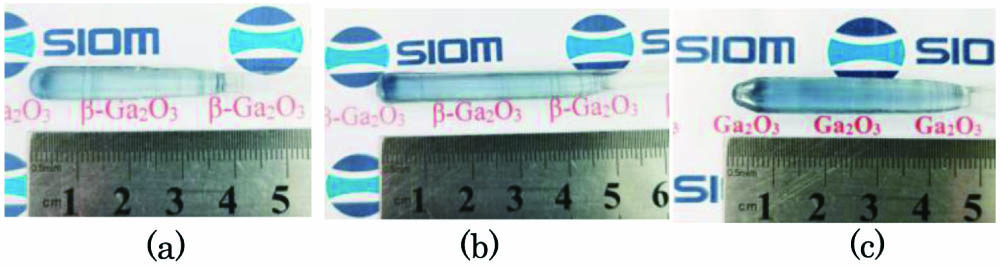
Set citation alerts for the article
Please enter your email address