Fig. 1. Schematic function demonstration of the proposed complex-amplitude RA-M. Beams with different characteristics can be obtained based on the proposed strategy. The inset illustrates the detailed structure of the meta-radiator in the dashed box.
Fig. 2. (a) Schematic principle of the complex-amplitude modulation capability. (b) Schematic diagram of the meta-radiator. (c) Simulated radiation amplitude and radiation phase at 10 GHz when the single ESRR rotates from 0° to 360° and the inset shows the surface current distribution of the single ESRR. (d) and (e) Simulated complex-amplitude modulation of dual SRRs with different rotation angles φ1 and φ2 at 10 GHz, where (d) presents the radiation amplitude ranging from 0 to 1 when Φsum is fixed and Φdiff changes from 0° to 90°, and (e) presents the radiation amplitude ranging from −180° to 180° when Φdiff is fixed and Φsum changes from 0° to 360°. The black dotted line represents the theoretically calculated value.
Fig. 3. Principle of the proposed far-field complex-amplitude retrieval (FCAR) method: the complex-amplitude iteration (CAI) consists of two processes, amplitude iteration (AI) and phase iteration (PI). The input information contains the preset of the scattered field plane and initial amplitude and phase in the aperture field plane. The initial value of amplitude or phase used in the PI or AI is the final value obtained during the previous iteration and remains constant throughout the iterative process. SSE is used as a criterion to judge the degree of convergence of the iteration. Here, the schematic diagram of the first CAI and the evolution of the scattered field are provided.
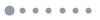
Fig. 4. Performance of the functional devices. The 2D patterns of the simulation results are given, and the inset gives the 3D patterns of the calculated results. In particular, the distribution of amplitude, phase, and the rotation angles of Case 1 is added. Energy-controllable multi-router: (a) two channels show a 44° beam with 0 dB and a −15° beam with −6.2 dB; (b) two channels show a 69° beam with 0 dB and a −10° beam with −3.1 dB; (c) four channels show −35° with −4.2 dB, −20° with 0 dB, 40° with −0.8 dB, 58° with −3.8 dB; (d) four channels show −50° with 0 dB, −19° with −4.2 dB, 39° with −4.9 dB, 59° with −0.2 dB; (e), (f) and (i), (j) the distribution of amplitude, phase, and the rotation angles of Case 1. Width-editable beams generator: (g) 40° fan beam ranges from −32° to 11°; (h) 25° fan beam ranges from −24° to 1°, 20° pencil beam with −0.79 dB intensity, 40° pencil beam with −3.79 dB, and 55° pencil beam with −7.1 dB. Tailorable beamformer: (k) −35° beam with −1.9 dB and 15° beam with 0 dB in the φ=0° plane; (l) 25° fan beam ranges from −19° to 11° in the φ=90° plane.
Fig. 5. (a) Schematic of the experimental setup for far-field measurement and the front and back views of fabricated RA-M’s photographs. (b) and (e) The 2D simulation (solid black) and measurement (red dashed) far-field results of four-channel energy-allocable multi-router named Sample I. (c) and (f) The 2D simulation and measurement far-field results of shape-editable beams generator named Sample II. (d) and (g) The 2D simulation and measurement far-field results of complex beamformer named Sample III. Black line indicates simulation results, and red dashed line indicates experimental results.
Fig. 6. (a) Simulated radiation amplitude of S11 when the single ESRR rotates from 0° to 330°. (b) and (c) The dimensions Φsum and Φdiff correspond to the radiation amplitude and radiation phase at 10 GHz.
Fig. 7. (a)–(c) Schematic diagram of the Floquet boundary conditions setting and surface currents distribution of Case 1–Case 3 when ESRR1 is excited. (d)–(f) S11 and S21 of Case 1–Case 3.
Fig. 8. Broadband radiation amplitude and phase characteristics of the two ESRRs. (a)–(d) Broadband radiation amplitude ranging from 9 to 11 GHz when Φsum is fixed. (e)–(h) Broadband radiation phase ranging from 9 to 11 GHz when Φdiff is fixed.
Fig. 9. 2D far-field patterns of four two-channel energy-allocable routers and four shape-editable beams generators are given; the inset gives the 3D patterns of the calculated results.
Fig. 10. Simulated amplitude of S11 of four two-channel energy-allocable routers and four shape-editable beams generators.
Fig. 11. Effect of array size on fan beam where the array size changes from 8×8 to 32×32 and the beam width changes from 20° to 80°. (a)–(d) The array size is fixed and the beam width is varied; (e)–(h) the beam width is fixed and the array size is varied.
Fig. 12. Simulated and measured gain characteristics of Sample I–Sample III.