
- Chinese Optics Letters
- Vol. 20, Issue 3, 031407 (2022)
Abstract
1. Introduction
Lasers, which implement retroreflectors as opposed to conventional cavity mirrors, have the potential of being alignment-free over long working distances[
As detailed in our former work and work by other groups, conventional cat-eye retroreflector designs typically comprise a focusing lens and a mirror positioned at its focal plane[
Figure 1.(a) Schematic of the alignment-free laser; (b) Zemax ray-trace model of the multi-lens retroreflector (the AoI interval between adjacent beams is 5°); and (c) the assembly drawing of the receiver lens tube.
2. Cavity and Optical Design
The schematic of the alignment-free distributed-cavity laser is shown in Fig. 1(a). The details of the transmitter (from the fiber-coupled diode pump to the intracavity lens F3) can be found in our prior work[
Sign up for Chinese Optics Letters TOC. Get the latest issue of Chinese Optics Letters delivered right to you!Sign up now
The distances between the four elements above (referred to as
The parameters of the lenses and mirror used to make up the retroreflector were optimized to provide good retroreflection for incident beams across a large FoV of
Diameter (mm) | Distance/Spacing (mm) | |||
---|---|---|---|---|
F4-1 | Plano-convex | 76.0/39.28 | 25.0 | |
F4-2 | Plano-concave | −160.0/−82.62 | 25.0 | |
F4-3 | Plano-convex | 25.4/13.08 | 25.0 | |
M3 | Plano-concave | −63.0/−32.5 | 50.0 |
Table 1. The Elements Used in the Multi-Lens Retroreflector
As illustrated in our former work, the SA and FC are the main issues that limit the working distance and the FoV, respectively[
Figure 2.Calculated FC-induced defocusing in terms of millimeters and diopters.
Figure 3.Power transfer of the laser at the working distances of 2 m and 5 m (AoI = 0°). The lines are a guide to the eye.
3. Results and Discussion
We first characterized the laser output power at an AoI of 0°, i.e., the transmitter and receiver were parallel with one another and shared a common optical axis. The results are plotted in Fig. 3. The laser threshold was
Figure 4 shows the laser output power as a function of working distance
Figure 4.Laser output power as a function of working distance L6 under an incident pump power of 16.6 W, with the receiver optimized at the working distance 5 m and with the receiver optimized at each point. The lines are a guide to the eye.
The receiver FoV (
Figure 5.Receiver FoV measured at a working distance of 2 m and 5 m (with an incident pump power of 16.6 W). The lines are a guide to the eye.
4. Conclusions
In this work, we designed an improved multi-lens retroreflector and used it in a distributed-cavity, alignment-free laser for resonant beam charging applications. The retroreflector, which was composed of off-the-shelf elements, was designed with a large aperture-limited FoV and with SA and FC compensated so that the distributed-cavity laser could operate efficiently over a long working distance and FoV. Using the improved multi-lens retroreflector as the receiver, the laser output power fluctuation was below 10%, with the receiver moving across a working distance of 1–5 m and tilted over a large FoV of
References
[1] B. Singh, S. R. Daultabad, V. V. Subramaniam, A. Chakraborty. Performance of an 80 W copper vapor laser with “alignment free” unstable CAT-EYE resonator and other configurations using intra-cavity apertures. Opt. Commun., 281, 6080(2008).
[2] P. Tripathi, R. Lovberg. A 700 meter long mode-locked argon ion laser. IEEE J. Quantum Electron., 11, 881(1975).
[3] G. Linford, E. Peressini, W. Sooy, M. Spaeth. Very long lasers. Appl. Opt., 13, 379(1974).
[4] Y. Wang, T. Dai, X. Liu, Y. Ju, B. Yao. Dual-wavelength injection-seeded Q-switched Ho:YLF laser for CO2 differential absorption lidar application. Opt. Lett., 44, 6049(2019).
[5] E. Mehdizadeh, J. Lunine, G. Atkinson. Intracavity laser spectroscopy with an ion-doped, solid-state Tm3+:YAG laser. J. Quant. Spectrosc. Radiat. Transf., 68, 453(2001).
[6] Q. Zhang, W. Fang, Q. Liu, J. Wu, P. Xia, L. Yang. Distributed laser charging: a wireless power transfer approach. IEEE Internet Things J., 5, 3853(2018).
[7] J. Lim, T. S. Khwaja, J. Ha. Wireless optical power transfer system by spatial wavelength division and distributed laser cavity resonance. Opt. Express, 27, A924(2019).
[8] M. Xiong, M. Liu, Q. Jiang, J. Zhou, Q. Liu, H. Deng. Retro-reflective beam communications with spatially separated laser resonator. IEEE Trans. Wirel. Commun., 20, 4917(2021).
[9] G. Zhou, A. Alfrey, L. Casperson. Modes of a laser resonator with a retroreflecting corner cube mirror. Appl. Opt., 21, 1670(1982).
[10] Y. Tan, S. Zhang. Alignment-free He–Ne laser with folded cavity. Opt. Lasers Eng., 46, 578(2008).
[11] R. Della-Pergola, O. Aplert, O. Nahmias, V. Vaisleib. Spatially distributed laser resonator. U.S. patent(2017).
[12] O. Aplert, E. Ronen, O. Nahmias, O. R. Mor, L. Golan, R. Sagi. Distributed coupled resonator cavity. U.S. patent(2019).
[13] Q. Sheng, M. Wang, H. Ma, Y. Qi, J. Liu, D. Xu, W. Shi, J. Yao. Continuous-wave long-distributed-cavity laser using cat-eye retroreflectors. Opt. Express, 29, 34269(2021).
[14] Q. Sheng, M. Wang, H. Ma, Y. Qi, J. Liu, D. Xu, W. Shi, J. Yao. Enhancing the field of view of a distributed-cavity laser incorporating cat-eye optics by compensating the field-curvature. Opt. Laser Technol., 151, 108011(2022).
[15] B. Fermigier, G. Lucas-Leclin, J. Dupont, F. Plumelle, M. Houssin. Self-aligned external-cavity semiconductor lasers for high resolution spectroscopy. Opt. Commun., 153, 73(1998).
[16] Z. Xu, S. Zhang, W. Du, Y. Li. Misalignment sensitivity of the cat’s eye cavity He–Ne laser. Opt. Commun., 265, 270(2006).
[17] Z. Xu, S. Zhang, Y. Li, W. Du. Adjustment-free cat’s eye cavity He–Ne laser and its outstanding stability. Opt. Express, 13, 5565(2005).
[18] W. Wang, Y. Gao, D. Sun, X. Du, J. Guo, X. Liang. Adjustable-free and movable Nd:YVO4 thin disk laser based on the telecentric cat’s eye cavity. Chin. Opt. Lett., 19, 111403(2021).
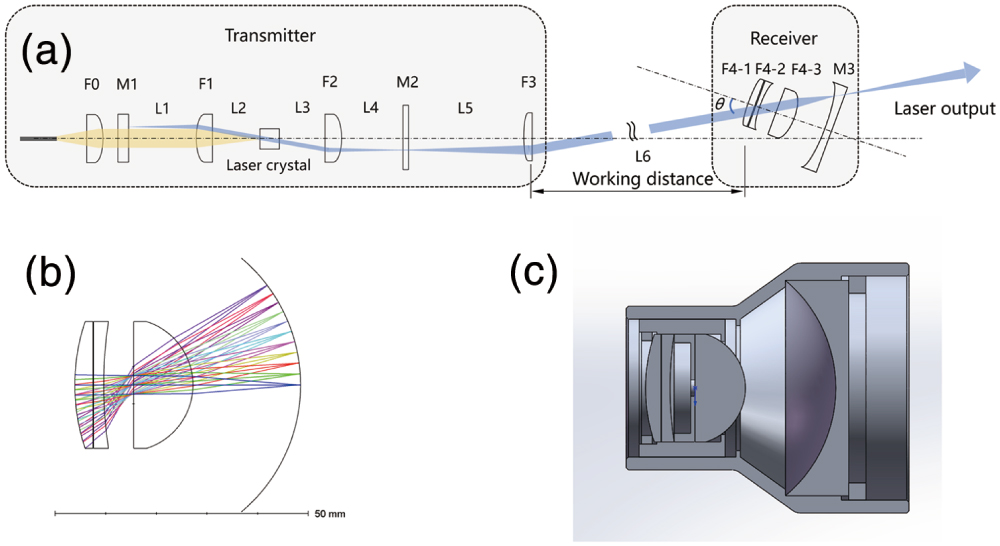
Set citation alerts for the article
Please enter your email address