
- Matter and Radiation at Extremes
- Vol. 7, Issue 6, 068102 (2022)
Abstract
I. THE UNEXPLOITED PRESSURE DIMENSION IN MATERIALS SCIENCE DUE TO LIMITATIONS OF HIGH-PRESSURE TECHNOLOGY
As a fundamental state variable, the application of pressure can dramatically alter the physics and chemistry of any material. In theory, extremely desirable material properties exist in the vast pressure range of hundreds of gigapascals (GPa) that is accessible using modern experimental techniques,1–3 but materials science currently only utilizes those properties that are available within the tiny slice near ambient pressure. High-pressure materials applications are currently restricted to the synthesis of a small number of quenchable phases that can be preserved in a metastable state for use under ambient conditions,4 and the extraordinary pressure-enhanced properties that vanish after decompression cannot yet be exploited.
The root of the problem is that GPa pressures are created by compressing a minuscule sample in a relatively gigantic pressure vessel, such as a diamond-anvil cell (DAC) or a multianvil press (MAP). Utilization of the pressure-enhanced properties of materials would require useful sample sizes that are comparable to that of the pressure vessel, as well as convenient access to the extraordinary pressure-induced properties through the vessel. To help achieve this goal, we can learn from natural mineral inclusions.
II. LEARNING FROM NATURAL AND SYNTHETIC MINERAL INCLUSIONS
Natural minerals crystallized from magma or hydrothermal fluid in the Earth’s deep interior can entrap foreign materials, such as fluids, other minerals, or rock fragments, to form inclusions. The host mineral completely encapsulates and isolates the inclusion from the surrounding environment. The host–inclusion relationship reveals valuable pressure–temperature–composition information about petrogenesis at the moment of lock-in.5 Nondestructive optical and x-ray techniques have been applied to probe mineral inclusions through the wall of the host mineral, and synthetic processes have been developed to simulate their conditions of formation.6 Diamond, the hardest known material,7 is capable of preserving the highest pressures in its inclusions. For instance, H2O with solid ice-VII structure at a pressure as high as 24 GPa has been discovered as an inclusion in diamond, thus providing direct evidence that the diamond originated from the lower mantle at 660 km depth.8
Generalizing this concept opens new perspectives for overcoming the aforementioned problems by synthesizing free-standing pressure-preserving capsules (PPCs). A successful example of the synthesis of free-standing nanocrystalline diamond capsules with crystalline argon inclusions preserved at 24 GPa after removal from a DAC has demonstrated the power of PPCs as a new approach to high-pressure science and technology.9
III. PERSPECTIVES FOR PPC SYNTHESIS
In principle, existing high-P–T apparatus, such as hydrothermal bombs, piston–cylinders, belts, toroidal presses, MAPs, and DACs, can be used to synthesize PPCs of micrometer to centimeter dimensions that preserve 1–100 GPa pressures. The PPC fixes its sample–envelope configuration at the high-P–T lock-in point, and a fraction of the sample pressure is preserved after the PPC has been quenched to ambient conditions according to the P–V–T equation-of-state difference between the sample and envelope.5,10 Diamond is an ideal envelope material owing to its unmatched strength and bulk modulus, but other superhard materials such as cubic boron nitride, cubic zirconia, and moissanite could also be used for specific applications. A variety of carbon allotropes, such as graphite, fullerenes, and glassy carbon, could be used as precursors for synthesizing diamond envelopes. The sample could be made of composite materials with an additional pressure medium or with additional envelopes to tailor the average bulk modulus of the sample assemblage or to avoid chemical incompatibility with the envelope. Free-standing PPCs could be further modified by surface machining while maintaining their integrity. Unlike conventional high-pressure apparatus, where pressure is a variable, each PPC represents a locked pressure point that only varies with temperature according to its thermoelastic behavior.5
IV. PERSPECTIVES FOR HIGH-PRESSURE SCIENTIFIC INVESTIGATIONS
Eliminating the restrictive requirement of an external pressure vessel enables the use of most modern probes for direct investigation of high-pressure phenomena in PPCs. The plethora of conventional high-pressure techniques for in situ optical spectroscopy, magnetic susceptibility, electrical resistivity, acoustic velocity, and x-ray diffraction, x-ray spectroscopy, and x-ray imaging studies, which have taken decades of effort to develop specifically for reaching high-pressure samples through the complicated geometry and containment of a pressure vessel, would now become straightforward in PPCs. For instance, synchrotron x-ray studies of PPCs would no longer require high-pressure beamlines dedicated to MAPs or DACs, but could be performed on general-purpose beamlines.
More importantly, with PPCs, it would be possible to use many powerful probes that are currently off-limits to high-pressure research. The possibility of reducing the PPC envelope to nanometer thickness opens the way for near-vacuum techniques, such as electron microscopy11 and vacuum ultraviolet to soft x-ray spectroscopy, to reach samples at high pressure. The micrometer to millimeter overall dimensions of PPCs makes high-pressure research accessible to techniques that can only be performed on small samples, such as magic-angle spin nuclear magnetic resonance (NMR), sub-millikelvin cryogenics, and the application of ultrahigh magnetic fields, thus extending the frontier of integration of pressure with other extreme conditions. The locked-pressure approach of PPCs also provides a perfect match with the single-shot operating mode at extremely powerful facilities such as those using pre-compressed targets for free-electron lasers and inertial confinement fusion.12 Exploration of the PPC technique will certainly have an across-the-board major impact on high-pressure scientific investigations.
V. PERSPECTIVES FOR HIGH-PRESSURE MATERIALS APPLICATIONS
Free-standing PPCs enable the exploitation of extremely favorable properties that exist only under high pressures.3 Functional materials can be pressure-tuned to their optimal performance in a superhard protective envelope that preserves the pressurized component while allowing the transmission of optical, thermal, electric, and magnetic energies, in a manner analogous to the glass envelope that separates the inner environment in a light bulb or vacuum tube while allowing the functional performance of the device. The feasible micrometer to centimeter dimensions and 1–100 GPa pressures attainable with PPCs are of practical relevance for use in both ordinary and nano devices. Given the high expense of making PPCs, at least at this initial stage, realistic applications should probably focus on high-value-added applications where greatly enhanced performance is the predominant concern. Examples include life-saving medical implant devices that need to last as long as possible, components for space missions that need maximized performance, and special devices that need to operate for extended periods in extreme environments.
ACKNOWLEDGMENTS
Acknowledgment. W.L.M. acknowledges support from NSF Geophysics Grant No. EAR 1446969. H.-K.M. is supported by the National Natural Science Foundation of China Grant No. U1930401.
References
[1] A. P.Drozdov, M. I.Eremets, V.Ksenofontov, S. I.Shylin, I. A.Troyan. Conventional superconductivity at 203 kelvin at high pressures in the sulfur hydride system. Nature, 525, 73-76(2015).
[2] I. A.Abrikosov, M. P.Belov, M.Bykov, S.Chariton, N.Dubrovinskaia, L.Dubrovinsky, T.Fedotenko, C.Giacobbe, S.Khandarkhaeva, D.Laniel, E.Lawrence Bright, A. V.Ponomareva, V.Prakapenka, P.Sedmak, N.Shulumba, E. A.Smirnova, F.Tasnádi, F.Trybel. Materials synthesis at terapascal static pressures. Nature, 605, 274-278(2022).
[3] J.Lv, Y.Ma, Y.Wang, L.Zhang. Materials discovery at high pressures. Nat. Rev. Mater., 2, 17005(2017).
[4] Y.Lin, W. L.Mao. Making the most of metastability. Science, 377, 814-815(2022).
[5] M.Alvaro, R. J.Angel, M. L.Mazzucchelli, F.Nestola, P.Nimis. Geobarometry from host-inclusion systems: The role of elastic relaxation. Am. Mineral., 99, 2146-2149(2014).
[6] J. D.Frantz, R. D.Giauque, K. W.Jones, H. K.Mao, M. L.Rivers, A. C.Thompson, J. H.Underwood, Y.Wu, Y.-G.Zhang. Analysis of fluid inclusions by X-ray fluorescence using synchrotron radiation. Chem. Geol., 69, 235-244(1988).
[7] Y.Tian, B.Xu. Diamond gets harder, tougher, and more deformable. Matter Radiat. Extremes, 5, 068103(2020).
[8] E.Greenberg, S.Huang, A.Lanzirotti, C.Ma, M.Newville, V. B.Prakapenka, G. R.Rossman, A. H.Shen, K.Tait, O.Tschauner, D.Zhang. Ice-VII inclusions in diamonds: Evidence for aqueous fluid in Earth’s deep mantle. Science, 359, 1136-1139(2018).
[9] B.Cheng, H.Lou, H.-k.Mao, W. L.Mao, L.Tan, J.Wen, L.Yang, W.Yang, Q.Zeng, Z.Zeng, X.Zhang, X.Zuo. Preservation of high-pressure volatiles in nanostructured diamond capsules. Nature, 608, 513-517(2022).
[10] B. A.Fursenko, S. V.Goryainov, R. J.Hemley, H.-k.Mao, J.Shu, N. V.Sobolev. Fossilized high pressure from the Earth’s deep interior: The coesite-in-diamond barometer. Proc. Natl. Acad. Sci. U. S. A., 97, 11875-11879(2000).
[11] S.Emmanuel, B.Jablon, O.Navon, C.Schmidt, A.Schreiber, R.Wirth. Solid molecular nitrogen (
[12] K.Lan. Dream fusion in octahedral spherical hohlraum. Matter Radiat. Extremes, 7, 055701(2022).
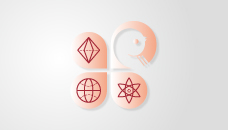
Set citation alerts for the article
Please enter your email address