
- Chinese Optics Letters
- Vol. 21, Issue 4, 041602 (2023)
Abstract
1. Introduction
The traditional optical data storage (ODS) materials are mainly azo metal chelates, cyanine dyes, and other organic dyes[1,2], which face considerable challenges due to poor physicochemical stability and low storage capacity. Therefore, it is of great significance to develop new optical storage media to advance the ODS applications. Electron-trapping materials (ETMs) have attracted a great deal of attention in the field of ODS due to their unique advantages of excellent rewritablity, environmental friendliness, high stability, and low cost. They are a kind of luminescent materials with light storage ability and delayed photon emissions under thermal stimulation or photostimulation[3]. It has a great application prospect in information storage[4–7], emergency display[8] and ex vivo bio-imaging[9–11].
Trap depth is one of the most important properties of ETMs, which affects the stability of optical information storage. However, the trap depth of ETMs could not meet the requirements for long-term information storage in previous research work. Hu et al. developed a novel phosphor
Yttrium aluminum garnet (
Sign up for Chinese Optics Letters TOC. Get the latest issue of Chinese Optics Letters delivered right to you!Sign up now
In this work, we prepared a series of
2. Experiments
2.1. Preparation of ceramics
Figure 1.Preparation flow chart of fluorescent ceramics. (a) Mixing and (b) ball milling and drying of raw materials, (c) filtering and shaping of samples, and (d) vacuum sintering of ceramics.
2.2. Material characterization
X-ray diffraction (XRD) patterns of samples were measured by using an X-ray diffractometer (Bruker D8 ADVANCE) with
3. Results and Analysis
3.1. Structure and optical properties
Figure 2 shows the XRD patterns of the
Figure 2.XRD patterns of YAG:xCe (x = 0.011, 0.013, 0.015, and 0.017) and Y3Al5O12 (PDF#33-0040).
As shown in Fig. 3(a), we tested the PLE spectrum (monitor wavelength:
Figure 3.(a) PLE and PL spectra of YAG:xCe (x = 0.011, 0.013, 0.015, and 0.017); (b) TL curves of YAG:xCe3+; (c) TL curves with different heating rates of YAG:0.015Ce3+; (d) ln(Tm2/β) versus 1/(kBTm) based on Eq. (
Trap levels are closely related to the information storage properties of ETMs. They are generally analyzed by the TL curve. TL involves the process in which storage optical information by irradiation with light is re-emitted by heating. As an effective tool to study the trap levels, the TL spectrum provides much information about the depth and amount of trap levels. Trap depth is one of the most important properties of ETMs; it is closely related to the stability of long-term storage of information. Therefore, we tested the TL spectra of the
There are two TL peaks in
3.2. Analysis of optical storage mechanism
We proposed the optical storage mechanism of the YAG:Ce, as shown in Fig. 4. In YAG:Ce,
Figure 4.Schematic of the optical storage mechanism of YAG:Ce.
3.3. Applications to ODS
As shown in Fig. 5(a), we have established a simple experimental scheme of ODS and readout to demonstrate the application in ODS. Under the cover of the photomask, the optical information “SIOM” is recorded in YAG:Ce ceramics by 254 nm Hg lamp and the information is readout by heating. Figure 5(b) panel (i) was taken under natural light. When exposed to 254 nm light, a green PL is produced from YAG:Ce [Fig. 5(b) panel (ii)]; it corresponds to the 5d-4f transition of
Figure 5.(a) Schematic illustration of the information storage and readout in ceramics; (b) photographs of YAG:Ce3+ ceramics under (i) natural light; (ii) 254 nm Hg UV light; (iii) and (iv) under 254 nm UV light for 5 min and heated to 300°C showing stars, flower, “S”, “I”, “O” and “M”, with photomask covering the sample.
4. Conclusions
In summary, we synthesized a series of fluorescent ceramics
References
[1] H. Mustroph, M. Stollenwerk, V. Bressau. Current developments in optical data storage with organic dyes. Angew. Chem. Int. Ed., 45, 2016(2006).
[2] M. Pawlicki, H. A. Collins, R. G. Denning, H. L. Anderson. Two-photon absorption and the design of two-photon dyes. Angew. Chem. Int. Ed., 48, 3244(2009).
[3] J. Xu, S. Tanabe. Persistent luminescence instead of phosphorescence: history, mechanism, and perspective. J. Lumin., 205, 581(2019).
[4] Y. Zhuang, D. Chen, W. Chen, W. Zhang, X. Su, R. Deng, Z. An, H. Chen, R. J. Xie. X-ray-charged bright persistent luminescence in NaYF4:Ln3+@NaYF4 nanoparticles for multidimensional optical information storage. Light Sci. Appl., 10, 132(2021).
[5] Q. Yang, R. Abdurahman, T. Yang, X. Sun. Wavelength-tunable barium gallate persistent luminescence phosphors with enhanced luminescence. Chin. Opt. Lett., 20, 031602(2022).
[6] S. Lin, H. Lin, C. Ma, Y. Cheng, S. Ye, F. Lin, R. Li, J. Xu, Y. Wang. High-security-level multi-dimensional optical storage medium: nanostructured glass embedded with LiGa5O8:Mn2+ with photostimulated luminescence. Light Sci. Appl., 9, 22(2020).
[7] W. Li, Y. Zhuang, P. Zheng, T. L. Zhou, J. Xu, J. Ueda, S. Tanabe, L. Wang, R. J. Xie. Tailoring trap depth and emission wavelength in Y3Al5-xGaxO12:Ce3+,V3+ phosphor-in-glass films for optical information storage. ACS Appl. Mater. Interfaces, 10, 27150(2018).
[8] J. Ueda, K. Kuroishi, S. Tanabe. Bright persistent ceramic phosphors of Ce3+-Cr3+-codoped garnet able to store by blue light. Appl. Phys. Lett., 104, 101904(2014).
[9] Z. Pan, Y. Y. Lu, F. Liu. Sunlight-activated long-persistent luminescence in the near-infrared from Cr3+-doped zinc gallogermanates. Nat. Mater., 11, 58(2012).
[10] Z. Liu, L. Zhao, W. Chen, X. Fan, X. Yang, S. Tian, X. Yu, J. Qiu, X. Xu. Multiple anti-counterfeiting realized in NaBaScSi2O7 with a single activator of Eu2+. J. Mater. Chem. C, 6, 11137(2018).
[11] J. Shi, X. Sun, J. Li, H. Man, J. Shen, Y. Yu, H. Zhang. Multifunctional near infrared-emitting long-persistence luminescent nanoprobes for drug delivery and targeted tumor imaging. Biomaterials, 37, 260(2015).
[12] C. Wang, Y. Jin, Y. Lv, G. Ju, D. Liu, L. Chen, Z. Li, Y. Hu. Trap distribution tailoring guided design of super-long-persistent phosphor Ba2SiO4:Eu2+,Ho3+ and photostimulable luminescence for optical information storage. J. Mater. Chem. C, 6, 6058(2018).
[13] H. Liu, B. Feng, L. Luo, C. Han, P. A. Tanner. Near infrared photostimulated persistent luminescence and information storage of SrAl2O4:Eu2+, Dy3+ phosphor. Opt. Mater. Express, 6, 3375(2016).
[14] M. Deng, Q. Liu, Y. Zhang, C. Wang, X. Guo, Z. Zhou, X. Xu. Novel co-doped Y2GeO5:Pr3+,Tb3+: deep trap level formation and analog binary optical storage with submicron information points. Adv. Opt. Mater., 9, 2002090(2021).
[15] S. Lin, H. Lin, Q. Huang, Y. Cheng, J. Xu, J. Wang, X. Xiang, C. Wang, L. Zhang, Y. Wang. A photostimulated BaSi2O5:Eu2+,Nd3+ phosphor-in-glass for erasable-rewritable optical storage medium. Laser Photonics Rev., 13, 1900006(2019).
[16] J. Li, Y. Wu, Y. Pan, W. Liu, L. Huang, J. Guo. Fabrication, microstructure and properties of highly transparent Nd:YAG laser ceramics. Opt. Mater., 31, 6(2008).
[17] J. Li, Y. Wu, Y. Pan, H. Kou, Y. Shi, J. Guo. Densification and microstructure evolution of Cr4+,Nd3+:YAG transparent ceramics for self-Q-switched laser. Ceram. Int., 34, 1675(2008).
[18] B. Yao, J. Yuan, J. Li, T. Dai, X. Duan, Y. Shen, Z. Cui, Y. Pan. High-power Cr2+:ZnS saturable absorber passively Q-switched Ho:YAG ceramic laser and its application to pumping of a mid-IR OPO. Opt. Lett., 40, 348(2015).
[19] R. Zhang, H. Lin, Y. Yu, D. Chen, J. Xu, Y. Wang. A new-generation color converter for high-power white LED: transparent Ce3+:YAG phosphor-in-glass. Laser Photonics Rev., 8, 158(2014).
[20] D. Chen, W. Xiang, X. Liang, J. Zhong, H. Yu, M. Ding, H. Lu, Z. Ji. Advances in transparent glass-ceramic phosphors for white light-emitting diodes: a review. J. Eur. Ceram. Soc., 35, 859(2015).
[21] B. Sun, B. Jiang, L. Zhang. Samarium and manganese incorporation to improve color rendering of LuAG:Ce3+ phosphor ceramics for laser-driven lighting: a color-tunable and energy transfer study. J. Mater. Chem. C, 9, 16468(2021).
[22] L. Wang, H. Yang, Y. Zhang, Y. Liang, J. Zhang, E. Mei, F. Xu, J. Long, P. Yu, W. Xiang. All-inorganic high efficiency LuAG:Ce3+ converter based on phosphor-in-glass for laser diode lighting. J. Alloy Compd., 892, 161882(2022).
[23] C. Wang, M. Deng, Y. Zhang, Q. Liu, Z. Zhou, X. Xu, H. He. Multicomponent garnet phosphor (LuYGd)(Al4Ga)O12:Ce3+,V3+: trap modulation via reductive gas annealing and an optical information storage property. Opt. Mater. Express, 11, 2256(2021).
[24] Y. Zhuang, Y. Lv, L. Wang, W. Chen, T. Zhou, T. Takeda, N. Hirosaki, R. Xie. Trap depth engineering of SrSi2O2N2:Ln2+,Ln3+(Ln2+ = Yb, Eu; Ln3+ = Dy, Ho, Er) persistent luminescence materials for information storage applications. ACS Appl. Mater. Interfaces, 10, 1854(2018).
[25] A. J. J. Bos. High sensitivity thermoluminescence dosimetry. Nucl. Instrum. Meth. B, 184, 3(2001).
[26] L. Yuan, Y. Jin, Y. Su, H. Wu, Y. Hu, S. Yang. Optically stimulated luminescence phosphors: principles, applications, and prospects. Laser Photonics Rev., 14, 2000123(2020).
[27] C. Wang, Y. Jin, J. Zhang, X. Li, H. Wu, R. Zhang, Q. Yao, Y. Hu. Linear charging-discharging of an ultralong UVA persistent phosphor for advanced optical data storage and wide-wavelength-range detector. Chem. Eng. J., 453, 139558(2023).
[28] Y. Jin, Y. Hu, L. Yuan, L. Chen, H. Wu, G. Ju, H. Duan, Z. Mu. Multifunctional near-infrared emitting Cr3+-doped Mg4Ga8Ge2O20 particles with long persistent and photostimulated persistent luminescence, and photochromic properties. J. Mater. Chem. C, 4, 6614(2016).
[29] D. Liu, L. Yuan, Y. Jin, H. Wu, Y. Lv, G. Xiong, G. Ju, L. Chen, S. Yang, Y. Hu. Tailoring multidimensional traps for rewritable multilevel optical data storage. ACS Appl. Mater. Interfaces, 11, 35023(2019).
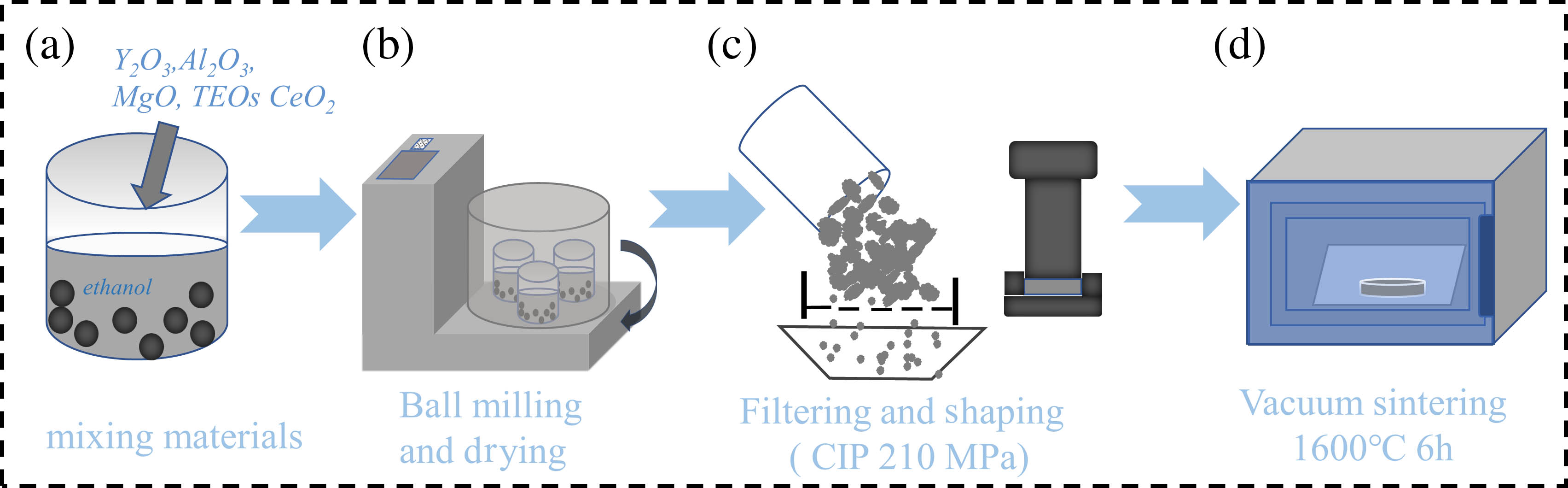
Set citation alerts for the article
Please enter your email address