Author Affiliations
1International Center for Computational Method and Software, College of Physics, Jilin University, Changchun 130012, People’s Republic of China2State Key Laboratory of Superhard Materials, College of Physics, Jilin University, Changchun 130012, People’s Republic of China3International Center of Future Science, Jilin University, Changchun 130012, People’s Republic of Chinashow less
A dream long held by physicists has been to raise the critical temperature (Tc)—the temperature below which the material exhibits no electrical resistance—of a superconductor to room temperature. The most recent excitement in that regard has centered on rare-earth superhydrides, of which LaH10 at 190 GPa has a remarkably high Tc of 260 K, approaching room temperature (Fig. 1)1–4 and originating from the appearance of a peculiar so-called “clathrate” structure in the crystal lattice that was reported for the first time in CaH6.5
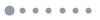
Figure 1.Chronological evolution of superconducting critical temperature (Tc) for various conventional superconductors.
The first hydride superconductor (Th4H15, Tc = 8 K) was discovered at ambient pressure in 1970.6 Importantly, that work initiated the search for superconductors among hydrogen-rich compounds as inspired by the proposal of high superconductivity in a metallic form of hydrogen, which is the lightest element, possibly giving rise to a high Debye temperature and a strong electron–phonon coupling necessary for a good phonon-mediated superconductor. Subsequent studies of Pd–H and Pd–Cu–H systems found Tc below 10 K. The low superconductivity is intimately related to the negligible contribution from hydrogen to the electronic density of states (EDOS) at the Fermi level (FL). After more than 30 years of silence, the search for superconductors among hydrogen-rich compounds was renewed by Ashcroft in 20047 via a “chemical pre-compression” effect that was proposed earlier by a pioneering theory in 1971.8
Superconductivity in hydrides is influenced by two key criteria, namely (i) a large hydrogen-derived EDOS at the FL and (ii) large modifications of the electronic structure in response to the vibration of hydrogen atoms,9 but the hydrogen-poor metal hydrides known at the time fit neither criteria. Ashcroft proposed the possibility of finding good superconductors among hydrides including those containing a rich amount of H2 molecules.7 However, once there is a stabilization of H2 molecules in the lattice, hydrides naturally fail to satisfy criterion (i) because hydrogen electrons in a major portion will occupy low-lying energy states far below the FL, arising from the formation of intramolecular H–H sigma bonds. There is a great wish to find hydrides containing as much atomic hydrogen as possible to satisfy both criteria and realize high-Tc superconductivity. Here, theory should play a leading role in aiding such a discovery.
It has long been believed that SH2 will dissociate into elemental hydrogen and sulfur under pressure. In 2014, this was revived by the appearance of theoretical work on the prediction of a superconductive structure (Tc = 80 K) at megabar pressures that is energetically stable with respect to elemental hydrogen and sulfur.10 Stimulated by this prediction, two distinct S–H superconductors were synthesized in experiments:11 (i) the one prepared at low temperature has Tc = 30 K–150 K, in accordance with the predicted SH2 superconductor;11 (ii) the one annealed at room temperature has an unexpectedly high Tc of 180 K–203 K, which is ascribed to a stoichiometric change from SH2 to SH3.9 Notably, SH3 is a known stoichiometry of (SH2)2H2 that has been synthesized at 7 GPa and is predicted to be a 200-K superconductor at megabar pressures.12 The finding of SH3 is a typical material-by-design success, with theory guiding the experimental discovery.
In 2012, a hitherto unknown superhydride of CaH6 with a remarkably high Tc of 235 K was predicted at megabar pressures in a Ca–H system via a structure-searching simulation.5 The high superconductivity arises from the peculiar clathrate structure comprising H24-cage units with encaged Ca.5 This CaH6 can be viewed as Ca-doped metallic hydrogen in which the hydrogen takes atomic form and contributes majorly to the EDOS at the FL. The clathrate structure is likely the first structural model for superhydrides in which hydrogen makes the dominant contribution to the EDOS at the FL. Subsequent structure-searching simulations revealed that clathrate structures in superhydrides are formed commonly in rare-earth superhydrides under pressure.1,2 Motivated by these theories, considerable experimental progress has been made, and the three best-known superconductors of LaH10, YH9, and YH6 were synthesized with Tc values of 260 K, 243 K, and 227 K, respectively.3,4,13 The finding of record-breaking superconductors among clathrate-structured rare-earth superhydrides highlights again the leading role of theory in the discovery.
A literature survey shows that nearly all binary hydrides have been investigated by structure-searching simulations. To date, the clathrate structure for metal superhydrides and the SH3 structure for covalent superhydrides are the two best structural models for hydride superconductors. To search further for a room-temperature superconductor, one must target ternary or quaternary superhydrides. So that the hydrides contain no H2 molecules, a useful strategy for achieving optimal superhydrides is to introduce extra electrons via metal doping into the lattice, thereby allowing the occupancy of H2 antibonding states for their dissociation; this is exemplified by a designed ternary Li2MgH16,14 mimicking the doping of Li into MgH16 whose lattice is rich in H2 molecules. This superhydride has the highest Tc of 473 K at 250 GPa and can be regarded as a hot superconductor. Indeed, recent experimental progress has shown high Tc values of 288 K15 and 550 K16 for C–S–H and La–B–N–H systems, respectively, but the information about compositions and structures is missing.
Although the present perspective gives several examples of theory-orientated discoveries of high-temperature superconductors in superhydrides, the main drawback of these materials is the destabilization at ambient pressure. There is a strong desire to discover high-Tc superhydrides that are quenchable at ambient pressure for practical use. However, direct synthesis at ambient pressure seems infeasible because there is a high energy gain for forming H2 molecules in the lattice. An alternative route is to seek synthesis at low pressures. In previous studies, we predicted that certain rare-earth superhydrides can be synthesized at moderate pressures, such as YbH6 at 40 GPa.1 With improved algorithms and computer power, structure-search computational schemes become extremely important aids for discovering new materials, and we expect theory-orientated discoveries of superconductors at room temperature or higher in superhydrides stabilized under pressure.
References
[1] C. J. Pickard, Y. Sun, Q. Wu, R. J. Needs, F. Peng, Y. Ma. Hydrogen clathrate structures in rare earth hydrides at high pressures: Possible route to room-temperature superconductivity. Phys. Rev. Lett., 119, 107001(2017).
[2] N. W. Ashcroft, R. Hoffmann, R. J. Hemley, I. I. Naumov, H. Liu. Potential high-Tc superconducting lanthanum and yttrium hydrides at high pressure. Proc. Natl. Acad. Sci. U. S. A., 114, 6990(2017).
[3] Y. Meng, V. V. Struzhkin, M. Baldini, Z. M. Geballe, M. Somayazulu, R. J. Hemley, M. Ahart, A. K. Mishra. Evidence for superconductivity above 260 K in lanthanum superhydride at megabar pressures. Phys. Rev. Lett., 122, 027001(2019).
[4] S. Mozaffari, M. Tkacz, L. Balicas, D. A. Knyazev, P. P. Kong, V. B. Prakapenka, D. E. Graf, S. P. Besedin, M. A. Kuzovnikov, V. S. Minkov, E. Greenberg, M. I. Eremets, A. P. Drozdov, F. F. Balakirev. Superconductivity at 250 K in lanthanum hydride under high pressures. Nature, 569, 528(2019).
[5] H. Wang, Y. Ma, J. S. Tse, K. Tanaka, T. Iitaka. Superconductive sodalite-like clathrate calcium hydride at high pressures. Proc. Natl. Acad. Sci. U. S. A., 109, 6463(2012).
[6] I. L. Toepke, C. B. Satterthwaite. Superconductivity of hydrides and deuterides of thorium. Phys. Rev. Lett., 25, 741(1970).
[7] N. W. Ashcroft. Hydrogen dominant metallic alloys: High temperature superconductors?. Phys. Rev. Lett., 92, 187002(2004).
[8] J. J. Gilman. Lithium dihydrogen fluoride—An approach to metallic hydrogen. Phys. Rev. Lett., 26, 546(1971).
[9] Y. Ma, Y. Wang, J. Lv, L. Zhang. Materials discovery at high pressures. Nat. Rev. Mater., 2, 17005(2017).
[10] Y. Li, J. Hao, H. Liu, Y. Ma, Y. Li. The metallization and superconductivity of dense hydrogen sulfide. J. Chem. Phys., 140, 174712(2014).
[11] V. Ksenofontov, M. I. Eremets, I. A. Troyan, A. P. Drozdov, S. I. Shylin. Conventional superconductivity at 203 kelvin at high pressures in the sulfur hydride system. Nature, 525, 73(2015).
[12] H. Yu, D. Li, D. Duan, Y. Liu, F. Tian, B. Liu, X. Huang, W. Tian, T. Cui, Z. Zhao. Pressure-induced metallization of dense (H2S)2H2 with high-Tc superconductivity. Sci. Rep., 4, 6968(2014).
[13] A. Sanna, J. A. Flores-Livas, G. Profeta, R. Arita, L. Boeri, M. Eremets. A perspective on conventional high-temperature superconductors at high pressure: Methods and materials. Phys. Rep., 856, 1-78(2020).
[14] Y. Xie, Y. Sun, Y. Ma, J. Lv, H. Liu. Route to a superconducting phase above room temperature in electron-doped hydride compounds under high pressure. Phys. Rev. Lett., 123, 097001(2019).
[15] A. Salamat, R. Mcbride, M. Debessai, E. Snider, N. Dasenbrock-Gammon, K. V. Lawler, K. Vencatasamy, R. P. Dias, H. Vindana. Room-temperature superconductivity in a carbonaceous sulfur hydride. Nature, 586, 373(2020).
[16] W. A. Coniglio, R. J. Hemley, S. W. Tozer, N. W. Ashcroft, M. Somayazulu, V. W. M. Oliff, A. D. Grockowiak, Y. Meng, T. Helm, M. Ahart, R. Kumar. Hot hydride superconductivity above 550 K(2020).