Dingpeng Liao, Fengliang Dong, Kun Zhang, Yi Zhou, Gaofeng Liang, Zhihai Zhang, Zhongquan Wen, Zhengguo Shang, Gang Chen, Luru Dai, Weiguo Chu, "Flat-field superoscillation metalens," Photonics Res. 10, 1924 (2022)

Search by keywords or author
- Photonics Research
- Vol. 10, Issue 8, 1924 (2022)
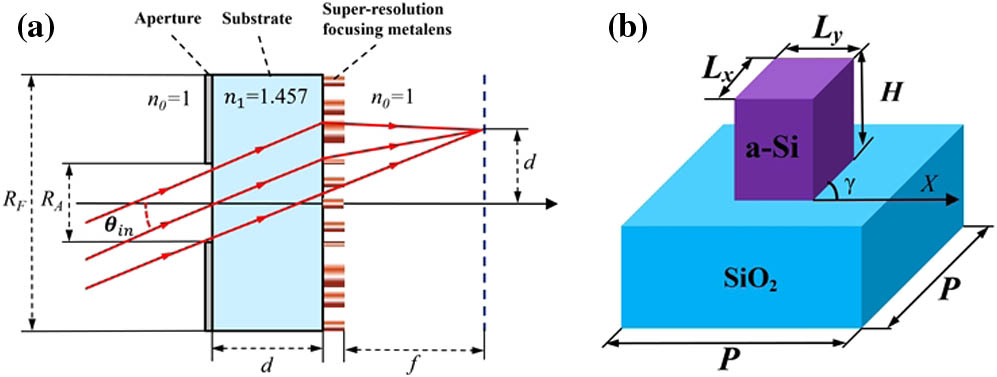
Fig. 1. Flat-field superoscillation metalens. (a) Schematic illustration of focusing of off-axis light by a flat-field superoscillation metalens. (b) Schematic illustration of the a-Si dielectric meta-atom for geometric-phase manipulation in the metalens.
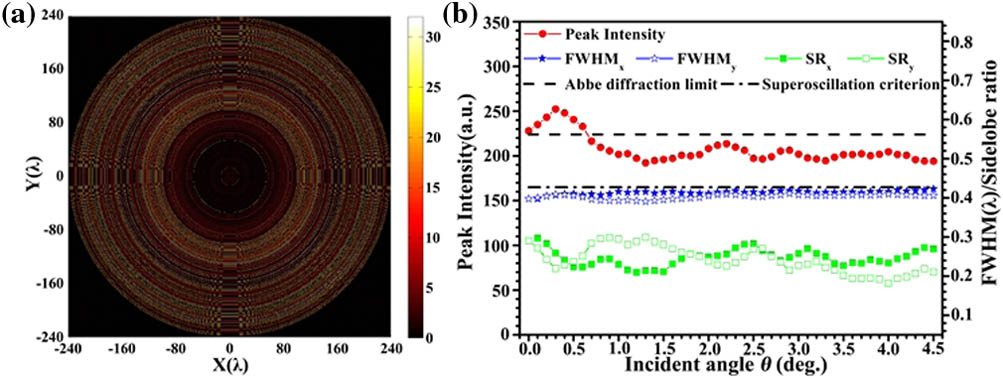
Fig. 2. Results of the optimized flat-field superoscillation metalens. (a) Phase profile of the lens φ ( x , y ) ; (b) peak intensity (red solid), full width at half-maximum FWHM x (blue solid) and FWHM y (blue open) in x and y directions, and sidelobe ratio in x (green solid) and y (green open) directions at different incident angles.
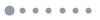
Fig. 3. (a) Diagram of the superoscillation focusing metalens arrangement in square lattice array, where inset gives the arrangement of the meta-atoms located at the device center. (b) SEM picture of the central part of the fabricated flat-field superoscillation metalens.
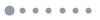
Fig. 4. Focusing performance of the flat-field superoscillation metalens. (a), (e) Simulation results of the optical intensity profile on the designed focal plane for different incident angles of 0°, 0.5°, 1.0°, 1.5°, 2.0°, 2.5°, 3.0°, 3.5°, 4.0°, and 4.5°; (b), (f) intensity distribution curves in x direction (blue) and y direction (red), both crossing the focal spot center; (c), (g) experimental results of the optical intensity profile on the designed focal plane for the 10 incident angles; (d), (h) intensity distribution curves in x direction (blue) and y direction (red), both crossing the focal spot center; (i) focusing performance parameters obtained from the experimental results, including peak intensity (red), spot size (FWHM) in x (blue solid) and y (blue open) directions and sidelobe ratio in x (green solid) and y (green open) directions; (j) spot displacement on the focal plane for the simulation result (red) and experimental result (blue).
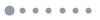
Fig. 5. Optical propagation properties on the incident plane (x z plane) at different incident angles. Simulation results: (a), (e) intensity distribution on x z propagation plane; (b), (f) peak intensity (red), FWHM (blue), and sidelobe ratio (green) along the propagation direction. Experimental results: (c), (g) intensity distribution on the x z propagation plane; (d), (h) peak intensity (red), FWHM (blue), and sidelobe ratio (green) along the propagation direction. The Abbe diffraction limit and superoscillation criterion are displayed by the dashed curve and dashed-dotted curve, respectively. The focal plane is denoted by the vertical dashed line.
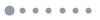
Fig. 6. Poynting vector on the incident plane (x z plane) at different incident angles, where the blue arrows give the direction of energy flow.
|
Table 1. Phase Distribution along Radial Direction
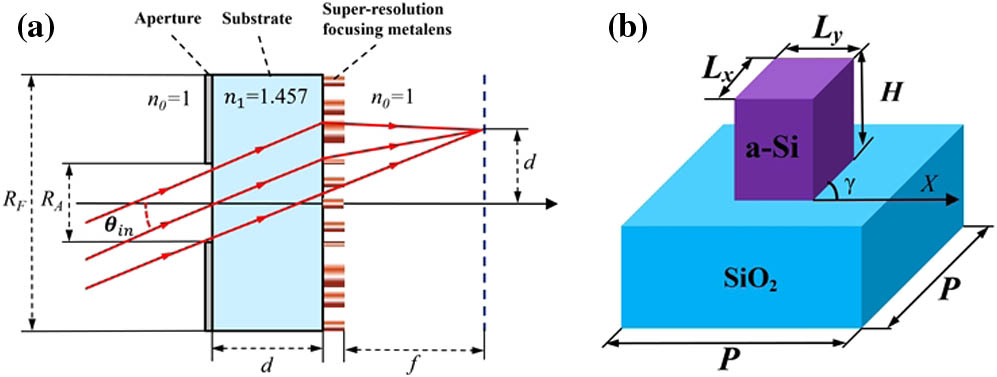
Set citation alerts for the article
Please enter your email address