Abstract
We experimentally demonstrate the generation of sub-100-fs pulses from a diode-pumped passively mode-locked (Yb:YSAG) ceramic laser. Stable mode-locked pulses as short as 96 fs at the central wavelength of 1052 nm with a repetition rate of are obtained. The laser has a maximum average output power of 51 mW. To the best of our knowledge, these are so far the shortest pulses and the first demonstration of sub-100-fs pulses obtained from the mode-locked Yb:YSAG ceramic lasers.Since the first demonstration of high-efficiency laser oscillation of the Nd:YAG ceramics in 1995, studies on ceramic laser materials and ceramic lasers have attracted considerable attention[1–16]. In comparison with single crystals and glasses, ceramic laser materials not only have good thermal, mechanical, and spectral properties, but also can be made in a large size, composite structures, and controlled dopant distributions. These advantages make the rare-earth-doped transparent ceramics promising gain media for different lasers. So far, many rare-earth-doped ceramic lasers have been reported with the same or even higher continuous-wave (CW) laser operation efficiency than that of the single crystals[2–11]. In the mode-locking regime, ultrashort pulse ceramic lasers have also attracted considerable interest. Mode-locking of a ceramic laser was first demonstrated by Shirakawa et al. in 2003[12]. They achieved stable mode-locked pulses with a 615 fs pulse width and 420 mW average power in a diode-pumped ceramic laser. Subsequently, they further demonstrated Kerr-lens mode-locking of diode-pumped and ceramic lasers[13,14] and achieved sub-100-fs mode-locked pulses among the ceramic lasers. In the meantime, mode-locking of the -doped garnet ceramic lasers has also been intensively investigated. Saikawa et al. first, to the best of our knowledge, demonstrated the passive mode-locking of a 15 at.% (Yb:YSAG) ceramic chip in 2004, which generated 280 fs pulses with an maximum average output power of 62 mW[15]. Afterward, mode-locking of the Yb:YAG and Yb:LuAG ceramic lasers was demonstrated in 2009 and 2012, generating ultrashort pulses of 233 and 541 fs with average output powers of 20 and 53 mW, respectively[16,17]. However, due to the relatively sharp and narrow gain bandwidth at around 1030 nm, it is challenging to obtain sub-100-fs ultrashort pulses for the -doped garnet materials. Only employing the Kerr-lens mode-locking technique mode-locked pulses with less than 100 fs were obtained in the -doped garnets[18–20].
A technique to broaden the gain bandwidth of rare-earth-doped garnets is to engineer the crystal properties through crystal mixing or disordering. Using the crystal engineering technique, even sub-50-fs pulses have been successfully achieved in [21], [22], [23], and [24] discorded crystal lasers. It is therefore to be expected that, based on the rare-earth-doped disordered ceramics, ultrashort mode-locked pulses could also be achieved in the -doped garnet ceramic lasers. The first, to the best of our knowledge, demonstration of -doped disordered transparent ceramic material was the Yb:YSAG ceramic fabricated by Saikawa et al. in 2004[25]. Also, previous studies on the Yb:YSAG ceramic have shown that it has a broader gain bandwidth than that of the Yb:YAG ceramics[15,25], implying it could be a good candidate to generate ultrashort pulses among the family of -doped garnet materials.
In this Letter, we report on the sub-100-fs mode-locked pulse emission of a diode-pumped Yb:YSAG disordered garnet ceramic laser. The absorption and emission spectra, as well as the wavelength tuning characteristic of a Yb:YSAG disordered ceramic laser, were investigated. The CW operation wavelength of the laser had a tunable range from 1029 to 1073 nm. Using a semiconductor saturable absorber mirror (SESAM) as the mode locker, stable CW mode-locked pulses with pulse duration of 96 fs and pulse repetition rate of at the center wavelength around 1052 nm were obtained. To the best of our knowledge, these are so far the shortest pulses and, also, the first demonstration of sub-100-fs pulses obtained from the mode-locked Yb:YSAG ceramic lasers.
Sign up for Chinese Optics Letters TOC. Get the latest issue of Chinese Optics Letters delivered right to you!Sign up now
The Yb:YSAG ceramic used in our experiment was fabricated by solid-state reactive sintering of high-purity powders of , , , and . It has a -doping concentration of 10 at.%. The absorption spectrum of the Yb:YSAG ceramic from 800 to 1100 nm at room temperature is shown in Fig. 1(a). There are three strong absorption peaks located at 913, 942, and 969 nm (zero-phonon line), respectively, which well match the emission wavelengths of the commercial high-power AlGaAs laser diodes (LDs). Especially, the broad absorption peak around 942 nm is very suitable for LD pumping. Figure 1(b) shows the room temperature emission spectra of the Yb:YSAG and Yb:YAG ceramics. Although the emission spectra of the two laser ceramics are similar, it can be identified that the two main emission peaks of the Yb:YSAG ceramic located at 1031 and 1050 nm are slightly red-shifted from the emission peaks of the Yb:YAG ceramic at 1030 and 1048 nm, respectively. In addition, the Yb:YSAG ceramic obviously has a broad emission bandwidth of , which is broader than that of the Yb:YAG ceramic[25]. The wide emission bandwidth indicates that the Yb:YSAG disordered garnet ceramic can potentially generate ultrashort mode-locked pulses.
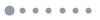
Figure 1.(Color online) (a) Absorption spectrum of a 10 at.% Yb:YSAG ceramic at room temperature and (b) emission spectra of 10 at.% Yb:YSAG and Yb:YAG ceramics.
First, the wavelength tuning performance of the Yb:YSAG ceramic laser under CW operation was investigated. An uncoated 10 at.% Yb:YSAG ceramic plate (16.4 mm in diameter and 2.4 mm in thickness) was cut into a sample of 4-mm-long and in a cross-section. The ceramic sample was Brewster cut with two laser-grade-polished end faces to minimize the transmission losses. To remove the generated heat under pumping and maintain the stable laser operation, the ceramic sample was wrapped with indium foil and tightly mounted in a copper heat sink block, which was cooled by circulating water. A fiber-coupled 940 nm LD was used as the pump source, and an X-folded cavity with an output coupler (OC) of at 1000–1100 nm was employed. A single SF10 prism was inserted at the Brewster angle in the arm with the OC as the intracavity wavelength tuning element. By changing the orientation of the OC, the emission wavelength and output power of the laser could be tuned. Also, a 44 nm broad tuning range from 1029 to 1073 nm was achieved under CW operation, indicating that the Yb:YSAG ceramic is able to generate short pulses under the mode-locking operation, as shown in Fig. 2. Moreover, it is found that relatively higher output power could be obtained near 1051 nm, while below 1031 nm the laser emission is strongly suppressed due to the reabsorption of ion. The wavelength tuning characteristic indicates that short pulse mode-locking would most probably occur near 1051 nm in the laser.
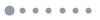
Figure 2.Wavelength tuning of the Yb:YSAG ceramic laser under CW operation with a 0.4% OC.
The experimental setup of the mode-locked Yb:YSAG ceramic laser was sketched in Fig. 3. An X-folded five-mirror cavity was used to achieve a suitable laser mode size on the SESAM and in the ceramic sample in the experiment. The Yb:YSAG ceramic was positioned between the M1 and M2 mirrors, which had the same radius of curvature (ROC) of 100 mm. The three dichroic concave mirrors M1, M2, and M3 were all high reflection coated in the wavelength range from 1020 to 1200 nm and anti-reflection coated from 808 to 980 nm. The 940 nm pump light was delivered by a multimode fiber, which has a core diameter of 105 μm and an NA of 0.22. After being collimated by an 80 mm focal length convex lens F1, the pump light was focused into the ceramic sample with a spherical convex lens F2, which had a focal length of 75 mm. Based on the ABCD matrix, the waist radius of the laser mode at the position of the laser gain medium and on the SESAM were estimated to be about 30 and 65 μm. To achieve mode-locking operation, a commercial SESAM (BATOP GmBH) with a modulation depth of 0.4%, non-saturable absorption of 0.3%, and recovery time of 1.0 ps at 1064 nm was employed as the mode locker. The OC used in the experiment had a transmission of 0.4% for the laser wavelength. To compensate the cavity group-delay dispersion (GDD) and achieve femtosecond operation, a pair of SF10 prisms with a tip-to-tip distance of 39 cm was used in the cavity, providing a negative dispersion value of about per round-trip.
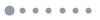
Figure 3.Experimental setup of the mode-locked Yb:YSAG ceramic laser. F1 and F2, convex lens, , ; M1, M2, and M3, concave mirror, .
As the absorbed pump power increased, the laser initially operated in the CW regime, and then -switching and the -switched mode-locking operation were achieved consecutively in the experiment. When the absorbed pump power exceeded 4.4 W, stable CW mode-locked operation was obtained. The average output power of the laser at the CW mode-locking threshold is about 30 mW. By slightly increasing the pump power and carefully optimizing the laser cavity, the mode-locked pulse spectrum could be remarkably broadened under the stable CW mode-locking operation regime. The mode-locked pulses were measured with a high-speed photodetector and displayed on a digital oscilloscope with 1.0 GHz bandwidth. Figure 4 shows the typical CW mode-locked pulse trains at 10 ns/div and 10 ms/div time scales, respectively. It can be found that the repetition rate of the pulses was about 102 MHz, corresponding to laser cavity length. The mode-locking was very stable and could be sustained for hours, where no -switched instability was observed in the experiment. When the incident pump power increased to about 6.7 W, mode-locked pulses as short as 96 fs were obtained in the experiment, corresponding to a maximum average output power of 51 mW. The mode-locking became unstable when the pump power was further increased. Due to the poor mode-matching between focused pump beam spot size ( in radius) and laser beam spot size ( in radius), a slit along the horizontal direction was placed close to the OC to suppress the possible high-order transverse mode oscillation of the laser, as shown in Fig. 3. In addition, to achieve fundamental transverse electromagnetic mode () operation and ultrashort pulses output, the laser cavity was also a little misaligned. These methods and low transmission of the OC resulted in the low output power and poor laser efficiency in the experiment. We also tried the Kerr-lens mode-locking by replacing the SESAM with a high reflective plane mirror, but there was no mode-locked signal observed.
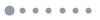
Figure 4.CW mode-locked pulse trains in nanosecond and millisecond time scales.
Figure 5(a) shows the autocorrelation trace of the mode-locked pulses measured by a commercial intensity autocorrelator (APE, PulseCheck 50). If assuming a -shaped pulse profile, the mode-locked pulses had a pulse width as short as 96 fs. Figure 5(b) shows the corresponding mode-locked spectra in linear (blue solid line) and logarithmic (red solid line) coordinates, which was measured with an optical spectrum analyzer (ANDO, AQ6315B). The mode-locked pulse spectra were centered at 1052 nm with a full width at half-maximum (FWHM) bandwidth of about 13.0 nm. The corresponding time-bandwidth product is calculated to be about 0.355, which is close to the Fourier transform limit of 0.315 for the -shaped pulses. Moreover, there is a strong peak at 1039.9 nm in the mode-locked spectra, which is a CW component that can be clearly identified experimentally. This CW component can be suppressed when the pump power decreased slightly, corresponding to the pulse width becoming a little wider at about 99 fs. Obvious Kelly sidebands can be observed on the mode-locked pulse spectra, especially in the logarithmic coordinate in Fig. 5(b). The appearance of Kelly sidebands, which were also observed in other sub-100-fs -doped lasers[20,21,24,26], is a clear signature of the soliton operation of mode-locked lasers. In the soliton operation regime, the spectrum of mode-locked pulses could be broadened by the strong self-phase modulation, and -shaped solitons are eventually formed due to the balance between the net negative cavity dispersion and the nonlinear self-phase modulation[27].
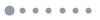
Figure 5.(Color online) (a) Autocorrelation trace and (b) the corresponding optical spectra of the mode-locked pulses.
In conclusion, we experimentally present a diode-pumped sub-100-fs Yb:YSAG disordered garnet ceramic oscillator. With aligning the cavity appropriately, stable CW mode-locked soliton pulses as short as 96 fs are successfully obtained when pumped by a multimode fiber-coupled diode laser. The mode-locked soliton pulses are centered at about 1052 nm with the maximum average output power of 51 mW and a pulse repetition rate of . To the best of our knowledge, these are so far the shortest pulses and also the first demonstration of sub-100-fs pulses obtained from the mode-locked Yb:YSAG ceramic lasers. Considering the ultrashort pulse formation in various disordered crystal lasers in recent years, we believe the disordered ceramic laser materials, which could have much better physical properties than the disordered crystals for laser applications, will attract more and more attention for generating high-power ultrashort pulses in various wavelength regions.
References
[1] A. Ikesue, T. Kinoshita, K. Kamata, K. Yoshida. J. Am. Ceram. Soc., 78, 1033(1995).
[2] A. Ikesue, Y. L. Aung, T. Taira, T. Kamimura, K. Yoshida, G. L. Messing. Ann. Rev. Mater. Res., 36, 397(2006).
[3] A. Ikesue, Y. L. Aung. Nat. Photon., 2, 721(2008).
[4] J. Lu, K.-I. Ueda, H. Yagi, T. Yanagitani, Y. Akiyama, A. A. Kaminskii. J. Alloy. Compd., 341, 220(2002).
[5] Y. Qi, X. Zhu, Q. Lou, J. Ji, J. Dong, Y. Wei. Opt. Express, 13, 8725(2005).
[6] X. Yang, H. Huang, D. Shen, H. Zhu, D. Tang. Chin. Opt. Lett., 12, 121405(2014).
[7] D. Luo, J. Zhang, C. Xu, H. Yang, H. Lin, H. Zhu, D. Tang. Opt. Mater. Express, 2, 1425(2012).
[8] A. V. Okishev. Opt. Lett., 37, 1199(2012).
[9] C. W. Xu, D. W. Luo, J. Zhang, H. Yang, X. P. Qin, W. D. Tan, D. Y. Tang. Laser Phys. Lett., 9, 30(2012).
[10] A. Pirri, D. Alderighi, G. Toci, M. Vannini. Opt. Express, 17, 23344(2009).
[11] J. Liu, L. Ge, L. Feng, H. Jiang, H. Su, T. Zhou, J. Wang, Q. Gao, J. Li. Chin. Opt. Lett., 14, 051404(2016).
[12] A. Shirakawa, K. Takaichi, H. Yagi, J. F. Bisson, J. Lu, M. Musha, K. Ueda, T. Yanagitani, T. S. Petrov, A. A. Kaminskii. Opt. Express, 11, 2911(2003).
[13] M. Tokurakawa, A. Shirakawa, K.-I. Ueda, H. Yagi, T. Yanagitani, A. A. Kaminskii. Opt. Lett., 32, 3382(2007).
[14] M. Tokurakawa, A. Shirakawa, K.-I. Ueda, H. Yagi, S. Hosokawa, T. Yanagitani, A. A. Kaminskii. Opt. Lett., 33, 1380(2008).
[15] J. Saikawa, Y. Sato, T. Taira, A. Ikesue. Appl. Phys. Lett., 85, 5845(2004).
[16] H. Yoshioka, S. Nakamura, T. Ogawa, S. Wada. Opt. Express, 17, 8919(2009).
[17] H. Nakao, A. Shirakawa, K.-I. Ueda, H. Yagi, T. Yanagitani. Opt. Express, 20, 15385(2012).
[18] U. Sadao, T. Kenji. Appl. Phys. Express, 1, 012007(2008).
[19] S. Uemura, K. Torizuka. Jpn. J. Appl. Phys., 50, 010201(2011).
[20] S. Kitajima, H. Nakao, A. Shirakawa, H. Yagi, T. Yanagitani. Opt. Lett., 41, 4570(2016).
[21] J. Ma, H. Huang, K. Ning, X. Xu, G. Xie, L. Qian, K. P. Loh, D. Tang. Opt. Lett., 41, 890(2016).
[22] P. Sévillano, P. Georges, F. Druon, D. Descamps, E. Cormier. Opt. Lett., 39, 6001(2014).
[23] A. Yoshida, A. Schmidt, V. Petrov, C. Fiebig, G. Erbert, J. Liu, H. Zhang, J. Wang, U. Griebner. Opt. Lett., 36, 4425(2011).
[24] J. Ma, H. Huang, H. Yu, H. Zhang, D. Tang. IEEE Photon. Technol. Lett, 28, 1298(2016).
[25] J. Saikawa, Y. Sato, T. Taira, A. Ikesue. Appl. Phys. Lett., 85, 1898(2004).
[26] J. Ma, J. Wang, D. Shen, H. Yu, H. Zhang, D. Tang. Opt. Express, 23, 32311(2015).
[27] O. E. Martinez, R. L. Fork, J. P. Gordon. Opt. Lett., 9, 156(1984).