
- Chinese Optics Letters
- Vol. 21, Issue 1, 011401 (2023)
Abstract
Keywords
1. Introduction
In the past two years, due to the global pandemic of coronavirus disease 2019 (COVID-19), ultraviolet C (UVC, 200–280 nm) disinfection technology to inactivate viruses effectively has attracted extensive attention at home and abroad. Compared to other disinfection methods such as chemical disinfection and high temperature disinfection, UVC disinfection has the advantages of rapid effectiveness, broad spectrum, thoroughness, no resistance to drugs, no secondary pollution, and so on[1]. At present, the ultraviolet (UV) light sources used typically for disinfection mainly include low-pressure mercury lamps and UV light-emitting diodes (UV-LEDs)[2,3]. The main emission line of the low-pressure mercury lamp is 253.7 nm[3], which is effective in killing bacteria, while for UV-LED is mainly from 265 to 280 nm[4,5]. However, all of these wavelengths of conventional UVC disinfection methods can penetrate the skin stratum corneum and the ocular tear layer. Therefore, these germicidal UVC lights will do damage to human health, and even cause skin cancer and cataracts[6,7]. In 2017, Buonanno et al.[8] firstly reported, to the best of our knowledge, that far-UVC light (200–225 nm) could kill bacteria effectively, where they used a krypton chloride (KrCl) excimer lamp that produced 222 nm UV light to destroy a variety of microbes. Some studies suggest[8–11] that far-UVC light has the same sterilization effect as conventional UVC light. Nevertheless, far-UVC light cannot penetrate the cuticle of human skin, so it is harmless to human body. In 2020, Buonanno et al.[12] used low doses of 222 nm UV light to inactivate human coronaviruses, which showed that 222 nm UV light could kill coronaviruses effectively without damaging human health. All of the above show that 222 nm UV disinfection technology is a promising tool for virus elimination.
At present, the most common and widely studied device for emerging far-UVC is a KrCl excimer lamp. The central wavelength of the excimer lamp is 222 nm, and there are two emission peaks of 235 nm and 325 nm[13]. Therefore, special optical filters are needed to filter the bands above 230 nm in order to avoid the adverse effects on human health. In 2018, Ruhnke et al.[14] used a 445 nm gallium nitride (GaN) external cavity laser diode (LD) frequency doubling to generate 222.5 nm UV laser output, where a narrowband deep UV laser with an output power of 160 µW was achieved. In China, as early as the end of the 1980s, nonlinear frequency conversion techniques were adopted to produce UV lasers. In 1992, Fan et al.[15] reported a 196–228 nm tunable UV laser, which is produced by frequency conversion of
The all-solid-state laser has the advantages of small size, long lifetime, high beam quality, and narrow linewidth[17,18]. Crucially, it can emit a single wavelength without the need of spectral purification. Therefore, UVC lasers generated by all-solid-state lasers based on nonlinear frequency conversion technology are a new trend in UVC light sources. Furthermore, all-solid-state lasers working in pulse mode can emit pulses with very short pulse width and high peak power. Compared with ordinary UV light, pulsed UV light has a faster sterilization rate because it has stronger penetration ability and can induce the destruction reaction of the virus molecular structure more quickly. Consequently, to achieve the same bactericidal effect, pulsed UV light takes only a few pulses to complete, while ordinary UV light requires much longer working time[19].
Sign up for Chinese Optics Letters TOC. Get the latest issue of Chinese Optics Letters delivered right to you!Sign up now
In this paper, a 222 nm all-solid-state far-UVC laser based on an optical parametric oscillator (OPO) and SHG was presented. Pumped by a home-made
2. Experimental Setup
The schematic diagram of the 222 nm all-solid-state far-UVC pulse laser system is shown in Fig. 1. The laser system is composed of three parts, which are a third-harmonic generation (THG)-based 355 nm UV laser unit, BBO-OPO unit, and UVC frequency doubling unit.
Figure 1.Schematic diagram of the experimental setup.
In the THG-based 355 nm laser unit, an LD pumped Nd:YAG laser operating at a repetition rate of 100 Hz offered fundamental laser pulses of 1064 nm, which were frequency-tripled to 355 nm by two
Sign up for Chinese Optics Letters TOC. Get the latest issue of Chinese Optics Letters delivered right to you!Sign up now
The OPO unit pumped by the 355 nm laser was a plane-concave resonator with the length of 55 mm. This cavity was composed of a plane mirror and a concave output coupler (OC). The plane mirror was high-reflection (HR) coated at 430–460 nm and anti-reflection (AR) coated at 355 nm and 1600–2000 nm, while the concave OC with the curvature radius of 1000 mm was partly reflection coated at 430–460 nm with transmission of 35% around 440 nm and AR coated at 355 nm and 1600–2000 nm. Two
The 444 nm laser beam from the OPO was then focused by convex lens
3. Results and Discussion
In this laser system, the Nd:YAG laser provided a maximum pulse energy of 10.3 mJ, and the pulse energy of 355 nm was around 4.37 mJ, the pulse width was about 10 ns, and the beam diameter was 1 mm. Consequently, the maximum peak-power density of the 355 nm laser was around
The signal output pulse energy of the OPO at 444 nm as a function of the pump pulse energy input is shown in Fig. 2. The output energy of the 444 nm laser increased linearly with the increasing pump energy of the 355 nm laser, and no saturation was observed. The maximum output pulse energy of 1.22 mJ at 444 nm wavelength was obtained with 4.37 mJ pump input, corresponding to a conversion efficiency of 27.9% and a slope efficiency of 39%.
Figure 2.Output pulse energy of the OPO at 444 nm as a function of the pump pulse energy.
The maximum conversion efficiency η in a singly resonant pulsed OPO is determined by[21]
Figure 3 shows the temporal profile of the 444 nm laser pulse output from this BBO OPO system, which was detected by Hamamatsu biplanar phototubes R1193U-52 and recorded by a Tektronix oscilloscope MDO4034C. The pulse duration of the signal at 444 nm was measured as 8.2 ns, which was less than 10 ns of the pump pulse duration at 355 nm.
Figure 3.Pulse temporal profile of the 444 nm laser.
The near-field beam profile of the 444 nm laser with a maximum output detected by a Spiricon silicon CCD camera (SP620) is shown in Fig. 4. The 444 nm laser beam was nearly circular and well-proportioned with the size about
Figure 4.Intensity distribution profile of 444 nm laser beam.
Figure 5.M2 factors measured for the 444 nm laser beam.
Figure 6 shows the output signal laser spectrum of the OPO, which was measured by the Yokogawa optical spectrum analyzer AQ6373B with the measurement resolution of 0.02 nm. The central wavelength was 444.01 nm, and the spectrum width was less than 0.05 nm.
Figure 6.Output laser spectrum of the OPO at 444 nm.
From the above results, it could be calculated that the peak-power density of the OPO output 444 nm laser was
Figure 7.Output pulse energy of SHG at 222 nm as a function of the fundamental pulse energy.
The spectrum of the 222 nm laser pulse was detected by an Ocean optic spectrometer (Maya2000Pro), as shown in Fig. 8. It could be read that the central wavelength of the SHG output laser was around 222.14 nm.
Figure 8.Spectrum of the 222 nm laser.
The pulse temporal profile of the 222 nm laser pulse was also detected by phototube R1193U-52 and recorded by a Tektronix oscilloscope MDO4034C, as shown in Fig. 9. The pulse duration was about 7.9 ns, which was a bit less than the fundamental pulse duration, and the peak-power density of 222 nm can be calculated as
Figure 9.Pulse temporal profile of the 222 nm laser.
Since a wide tuning range of the OPO can be achieved by symmetrically rotating those two BBO crystals, a tunable output in UVC was demonstrated in this experimental setup. Figure 10 shows the detected tuning output curve with a range of 42.5 nm from 210 nm to 252.5 nm, and the maximum UVC pulse energy was above 160 µJ.
Figure 10.Tunable output in UVC spectral range.
4. Conclusion
In summary, a 222 nm all-solid-state far-UVC laser based on BBO-OPO and BBO-SHG was demonstrated. At a pulse repetition rate of 100 Hz, with maximum pump pulse energy of 4.37 mJ at 355 nm, 1.22 mJ pulse energy at 444 nm wavelength was obtained from the BBO-OPO system, corresponding to a conversion efficiency of 27.9%, and, finally, 164.9 µJ pulse energy at the 222 nm wavelength was detected, corresponding to an SHG optical-to-optical conversion efficiency of 16.2%. The laser pulse energy density at 222 nm is sufficient for virus inactivation. Such a compact, high peak-power far-UVC laser has a great prospect for the applications of UV disinfection.
References
[1] B. Ma, P. M. Gundy, C. P. Gerba, M. D. Sobsey, K. G. Linden. UV inactivation of SARS–CoV–2 across the UVC spectrum: KrCl excimer, mercury-vapor, and light–emitting–diode (LED) sources. Appl. Environ. Microbiol., 87, e01532(2021).
[2] N. M. Hull, K. G. Linden. Synergy of MS2 disinfection by sequential exposure to tailored UV wavelengths. Water Res., 143, 292(2018).
[3] S. E. Beck, R. A. Rodriguez, K. G. Linden, T. M. Hargy, T. C. Larason, H. B. Wright. Wavelength dependent UV inactivation and DNA damage of adenovirus as measured by cell culture infectivity and long range quantitative PCR. Environ. Sci. Technol., 48, 591(2014).
[4] K. G. Linden, N. Hull, V. Speight. Thinking outside the treatment plant: UV for water distribution system disinfection. Acc. Chem. Res., 52, 1226(2019).
[5] S. E. Beck, H. Ryu, L. A. Boczek, J. L. Cashdollar, K. M. Jeanis, J. S. Rosenblum, O. R. Lawal, K. G. Linden. Evaluating UV-C LED disinfection performance and investigating potential dual-wavelength synergy. Water Res., 109, 207(2017).
[6] S. Zaffifina, V. Camisa, M. Lembo, M. R. Vinci, M. G. Tucci, M. Borra, A. Napolitano, V. Cannatã. Accidental exposure to UV radiation produced by germicidal lamp: case report and risk assessment. Photochem. Photobiol., 88, 1001(2012).
[7] R. B. Setlow, E. Grist, K. Thompson, A. D. Woodhead. Wavelengths effective in induction of malignant melanoma. Proc. Natl Acad. Sci. USA, 90, 6666(1993).
[8] M. Buonanno, B. Ponnaiya, D. Welch, M. Stanislauskas, G. Randers-Pehrson, L. Smilenov, F. D. Lowy, D. M. Owensb, D. J. Brennera. Germicidal efficacy and mammalian skin safety of 222–nm UV light. Radiat. Res., 187, 493(2017).
[9] M. Buonanno, G. Randers-Pehrson, A. W. Bigelow, S. Trivedi, F. D. Lowy, H. M. Spotnitz, S. M. Hammer, D. J. Brenner. 207–nm UV light–a promising tool for safe low-cost reduction of surgical site infections. I: in vitro studies. PLoS ONE, 8, e76968(2013).
[10] M. Buonanno, M. Stanislauskas, B. Ponnaiya, A. W. Bigelow, G. Randers-Pehrson, Y. Xu, I. Shuryak, L. Smilenov, D. M. Owens, D. J. Brenner. 207-nm UV light-a promising tool for safe low-cost reduction of surgical site infections. II: in vivo safety studies. PLoS ONE, 11, e0138418(2016).
[11] B. Ponnaiya, M. Buonanno, D. Welch, I. Shuryak, D. J. Brenner. Far–UVC light prevents MRSA infection of superficial wounds in vivo. PLoS ONE, 13, e0192053(2018).
[12] M. Buonanno, D. Welch, I. Shuryak, D. J. Brenner. Far–UVC light (222 nm) efficiently and safely inactivates airborne human coronaviruses. Sci. Rep., 10, 10285(2020).
[13] J. Zhanga, I. W. Boyd. Efficient excimer ultraviolet sources from a dielectric barrier discharge in rare-gas/halogen mixtures. J. Appl. Phys., 80, 633(1996).
[14] N. Ruhnke, A. Müller, B. Eppich, M. Maiwald, B. Sumpf, G. Erbert, G. Tränkle. Compact deep UV system at 222.5 nm based on frequency doubling of GaN laser diode emission. IEEE Photonics Technol. Lett., 30, 289(2018).
[15] Q. Fan, Z. Lu, B. Wu, Z. Hong. Generation of UV radiation of 196–228 nm with BBO crystal. Acta Opt. Sin., 12, 403(1992).
[16] X. Zhang, Z. Wang, G. Wang, Y. Zhu, Z. Xu, C. Chen. Widely tunable and high-average-power fourth-harmonic generation of a Ti:sapphire laser with a KBe2BO3F2 prism-coupled device. Opt. Lett., 34, 1342(2009).
[17] M. Zhao, C. Wang, Q. Hao, Z. Zou, J. Liu, X. Fan, L. Su. High single-pulse energy passively Q-switched laser based on Yb, Gd:SrF2 crystal. Chin. Opt. Lett., 18, 101401(2020).
[18] Y. Zhang, J. Zou, W. Zheng, K. Feng, B. Xu, Z. Yu. Watt-level continuous-wave intracavity frequency-doubled Pr:YLF-LBO laser at 320 nm. Chin. Opt. Lett., 19, 091406(2021).
[19] C. Wang, L. Hu, J. Long. The mechanism and application prospect of pulsed UV strong light sterilization technology. Chin. J. Disinfect., 33, 1104(2016).
[20] J. Zhang, J. Ma, T. Lu, D. Liu, X. Zhu, W. Chen. Compact wavelength tunable output around 440 nm pulsed laser for oceanic lidar application. Opt. Commun., 485, 126706(2021).
[21] E. Granot, S. Pearl, M. M. Tilleman. Analytical solution for a lossy singly resonant optical parametric oscillator. J. Opt. Soc. Am. B, 17, 381(2000).
[22] D. Welch, M. Buonanno, V. Grilj, I. Shuryak, C. Crickmore, A. W. Bigelow, G. Randers-Pehrson, G. W. Johnson, D. J. Brenner. Far-UVC light: a new tool to control the spread of airborne-mediated microbial diseases. Sci. Rep., 8, 2752(2018).
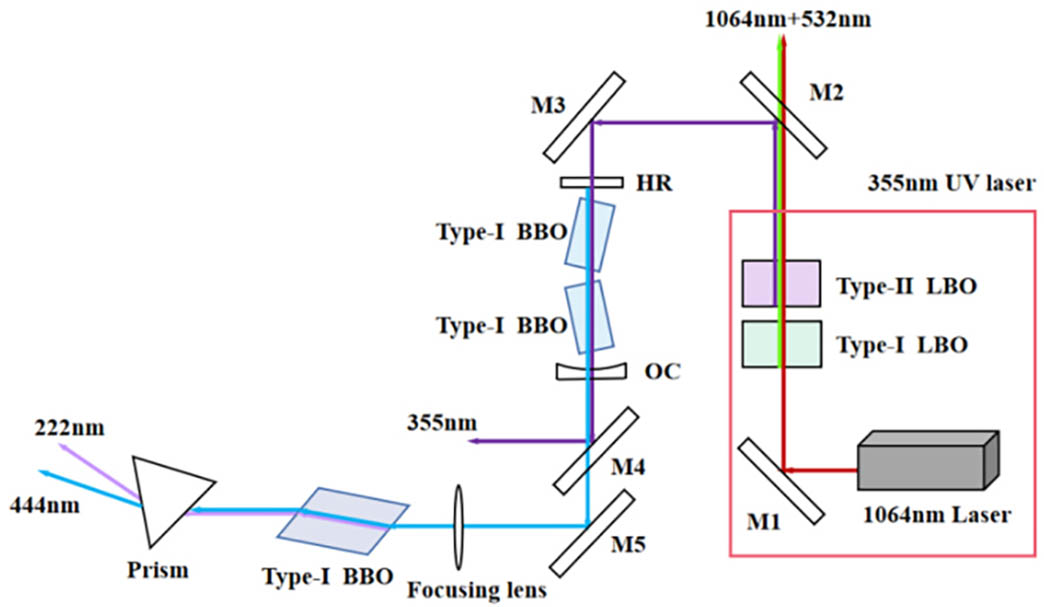
Set citation alerts for the article
Please enter your email address