
- Journal of Semiconductors
- Vol. 40, Issue 11, 111607 (2019)
Abstract
1. Introduction
As is known to us, skin plays an important role in protecting body from the external environment[
It is found that using appropriate wound dressing to cover the wound would accelerate the wound healing process[
Ideally, the wound monitoring technologies and strategies should be simple, rapid, precise, could be completed during a standard medical consultation and cause minimal or no discomfort to the patient[
2. pH in wound healing process
Generally, wound healing process is a dynamic process including inflammation, proliferation, and remodeling stages[
Figure 1.(Color online) Wound healing phases: (a) inflammation, (b) proliferation, and (c) remodeling. Reprinted with permission from Ref. [
3. pH sensors for wound monitoring
The pH notation is a measurement of H+ concentration and can be measured in the range of 0–14 on the negative logarithmic scale (pH = –log[H+])[
3.1. Flexible optical pH sensors for wound monitoring
One of the effective and simple methods for monitoring pH changing is using pH indicator dyes, which can absorb selected visible light wavelengths depending on the pH and then show naked eye color changing[
According to this approach, Trupp et al. have developed an optical pH sensor with synthesized hydroxyl-substituted azobenzene derivatives as the pH indicator dyes, and cellulose films as the substrate[
Figure 2.(Color online) (a) Flexible array-type pH sensor. (b) Color change on the pH from acidic to basic[
Similarly, Liu et al. have designed a pH sensitive hydrogel wound patch incorporated with the Phenol red (PR) dye modified with methacrylate (MA)[
To achieve the higher sensitivity and more effective composite wound dressing, pH sensitive electrospun nanofiberous films were developed[
More recently, an electrospun film is developed for monitoring the wound pH by loading pH-sensitive color changing curcumin into the polycaprolactone (PCL) matrix[
3.2. Wearable electrochemical pH sensors for wound monitoring
To develop more precise pH sensors, electrochemical techniques were employed to measure potential, current and so on for responding pH[
Wang et al. have developed a wearable electrochemical pH sensor based on bandages for monitoring the pH of a wound[
Figure 3.(Color online) (a) Fabrication process to create the pH-sensitive bandage. (b) A real-time pH changes in a simulated wound[
Similarly, Rahimi et al. designed an economical flexible pH array sensors based on a commercial paper (palettepaper) as illustrated in Fig. 3(c)[
3.3. Wireless communication for flexible and wearable pH sensors
Generally, for the optical flexible pH sensors, the change of the pH in wound site could be examined by naked eye through the color change, which is one of the effective and simple methods to show wireless communication for monitoring wound pH changing.
However, for the electrochemical pH sensors mentioned above, the pH signal must be measured and readout by specialized instruments, which limited these sensors for personalized application in home. To make the pH sensors more convenient for use, wireless technologies were employed on the fabrication of pH sensors[
Sridhar and Takahata have designed a hydrogel-based wireless pH sensor as shown in Fig. 4(a). It can be seen that this sensor has a sandwich structure with two aligned coils by folding a flexible conductive polyimide film forming a gap and a pH sensitive poly(vinyl alcohol)-poly(acrylic acid) (PVA-PAA) hydrogel in the middle to adjust the gap. Since the PVA-PAA hydrogel shows a pH relative volume/dimension change in different pH range from 2–7, the inductance of the coil could be changed, as well as the frequency changed along with the inductance. Additional with a designed wireless measurement device, an magnetically coupled external coil, the change of frequency depending on pH can be detected by a 4396B network-spectrum analyzer[
Figure 4.(Color online) (a) Design of a pH sensitive gel placed between two inductive coils. Relationship between the resonant frequency, the gap and the pH[
Following this way, Pal et al. have developed a low-cost, single-use, omniphobic paper-based smart bandages (OPSBs) to monitor the pH of open chronic wounds, as shown in Fig. 4(b)[
Furthermore, Rahimi et al. have presented a low-cost smartphone based pH sensing platform that consists of a flexible, disposable, and transparent pH sensor interfaced with a custom-designed flexible, battery-less, and reusable near-field communication (NFC) tag[
To take both the advantages of the optical and electrochemical pH sensors, kinds of sensors integrated with custom CMOS readout electronics for wireless monitoring wound pH and data transmission has been developed[
As shown in Fig. 5, Punjiya et al. have designed a wireless pH sensing bandage with numerous pH sensing smart threads for chronic wound monitoring[
Figure 5.(Color online) (a) Optical image of pH sensing bandage (left) and SEM of the pH sensitive threads. (b) Open circuit voltage versus pH for the threads. (c) Data acquisition setup for pH sensing smart bandage. (d) Smart bandage pH map[
4. Conclusion and future challenges
In conclusion, pH sensors for monitoring the wound healing have gained much attention in recent years. These sensors based on several pH sensitive mechanisms such as optical and electrochemical ones by providing different signals. Moreover, the reported pH sensors mostly based on flexible substrate such as polymer films, textiles and bandages to ensure their flexible and wearable properties. Furthermore, wireless transmission is also a popular trend for the development of these pH sensors, even can use a smart phone to collect the wound pH values and then determine the wound healing situation. While, there still some challenges for the pH sensors at the present stage.
(1) Development of large scale pH sensors for monitoring the large-area chronic wounds such as burn injury and diabetic foot wound. To produce large scale pH sensors, electrospinning may be a possible method to achieve it.
(2) Development of in situ fabrication of pH sensors incorporated with composite wound dressing directly onto the wound site. As the development of the in situ electrospinning technology through portable electrospinning devices and elctrospun pH nanofiber sensors, the composite nanofibrous wound dressing will be a new approach for wound healing.
(3) Development more precise optical pH sensors with naked eye color change. The color change should be more clear to be distinguished by the naked eye.
(4) pH sensors is a critical concern for wound monitoring, especially for directly contacting the wound. Researcher should pay attention on this point and develop the pH sensors using biocompatible materials.
Acknowledgments
This work was supported by the National Natural Science Foundation of China (No. 51703102), and the Innovation and Entrepreneurship Training Program for College Students of Qingdao University (2019).
References
[1] L Yildirimer, N T K Thanh, A M Seifalian. Skin regeneration scaffolds a multimodal bottom-up approach. Trends Biotechnol, 30, 638(2012).
[2] J R Dias, P L Granja, P J Bártolo. Advances in electrospun skin substitutes. Prog Mater Sci, 84, 314(2016).
[3]
[4] N Mehmood, A Hariz, R Fitridge et al. Applications of modern sensors and wireless technology in effective wound management. J Biomed Mater Res Part B, 102B, 885(2014).
[5] G C Gurtner, S Werner, Y Barrandon et al. Wound repair and regeneration. Nature, 453, 314(2008).
[6] M G Tonnesen, X Feng, R A Clark. Angiogenesis in wound healing. J Invest Dermatol Symp Proc, 5, 40(2000).
[7] N B Menke, K R Ward, T M Witten et al. Impaired wound healing. Clin Dermatol, 25, 19(2007).
[8] T Abdelrahman, n H Newton. Wound dressings: Principles and practice. Surgery (Oxford), 29, 491(2011).
[9] N Mao, S J Russell. Nonwoven wound dressings. Textil Progr, 36, 4, 1(2004).
[10]
[11] A J Bandodkar, J Wang. Non-invasive wearable electrochemical sensors: a review. Trends Biotechnol, 32, 363(2014).
[12] S Wang, T Chinnasamy, M A Lifson et al. Flexible substrate-based devices for point-of-care diagnostics. Trends Biotechnol, 34, 909(2016).
[13] E Gianino, C Miller, J Gilmore. Smart wound dressings for diabetic chronic wounds. Bioengineering, 5, 51(2018).
[14] M S Brown, B Ashley, A Koh. Wearable technology for chronic wound monitoring: current dressings, advancements, and future prospects. Front Bioeng Biotechnol, 6, 47(2018).
[15] M Ochoa, R Rahimi, B Ziaie. Flexible sensors for chronic wound management. IEEE Rev Biomed Eng, 7, 73(2014).
[16] X Wang, Z Liu, T Zhang. Flexible sensing electronics for wearable/attachable health monitoring. Small, 13, 1602790(2017).
[17] Q Sun, B Qian, K Uto et al. Functional biomaterials towards flexible electronics and sensors. Biosens Bioelectron, 119, 237(2018).
[18] L A Schneider, A K S Grabbe, J Dissemond. Influence of pH on wound-healing: a new perspective for wound-therapy. Arch Dermatol Res, 298, 413(2007).
[19] G Gethin. The significance of surface pH in chronic wounds. Wounds UK, 3, 3(2007).
[20] V K Shukla, D Shukla, S K Tiwary et al. Evaluation of pH measurement as a method of wound assessment. J Wound Care, 16, 7(2007).
[21] S Schreml, R M Szeimies, S Karrer et al. The impact of the pH value on skin integrity and cutaneous wound healing. J Eur Acad Dermatol, 24, 373(2010).
[22] S Percival, S McCarty, J Hunt et al. The effects of pH on wound healing, biofilms, and antimicrobial efficacy. Wound Repair Regen, 22, 174(2014).
[23] S Lei, R Sarah, E Ryan et al. pH in the bacteria-contaminated wound and its impact on clostridium histolyticum collagenase activity: implications for the use of collagenase wound debridement agents. J Wound Ostomy Cont, 38, 514(2011).
[24] M A Khan, U U Ansari, M N Ali. Real-time wound management through integrated pH sensors: a review. Sens Rev, 35, 183(2015).
[25] C Schaude, E Fröhlich, C Meindl et al. The development of indicator cotton swabs for the detection of pH in wounds. Sensors, 17, 1365(2017).
[26] S M Al-Hillia, M Willander, A Öst et al. ZnO nanorods as an intracellular sensor for pH measurements. J Appl Phys, 102, 084304(2007).
[27] T R Dargaville, B L Farrugia, J A Broadbent et al. Sensors and imaging for wound healing: a review. Biosens Bioelectron, 41, 30(2013).
[28]
[29] A U Alam, Y Qin, S Nambiar et al. Polymers and organic materials-based pH sensors for healthcare applications. Prog Mater Sci, 96, 174(2018).
[30] D Wencel, T Abel, C McDonagh. Optical chemical pH sensors. Anal Chem, 86, 15(2014).
[31] Y Miao, J Chen, K Fang. New technology for the detection of Ph. J Biochem Biophy. Methods, 63, 1(2005).
[32]
[33] L V Der Schueren, K D Clerck. Coloration and application of pH-sensitive dyes on textile materials. Color Technol, 128, 82(2012).
[34] L V Der Schueren, K D Clerck. The use of pH-indicator dyes for pH-sensitive textile. Text Res J, 80, 590(2010).
[35] S Trupp, M Alberti, T Carofiglio et al. Development of pH-sensitive indicator dyes for the preparation of micro-patterned optical sensor layers. Sens Actuators B, 150, 206(2010).
[36] G J Mohr, H Müller, B Bussemer et al. Design of acidochromic dyes for facile preparation of pH sensor layers. Anal Bioanal Chem, 392, 1411(2008).
[37]
[38] L Liu, X Li, M Nagao et al. A pH-Indicating colorimetric tough hydrogel patch towards applications in a substrate for smart wound dressings. Polymers, 9, 558(2017).
[39] Schueren L V der, Mollet T T, Ö Ceylan et al. The development of polyamide 6.6 nanofibres with a pH-sensitive function by electrospinning. Eur Polym J, 46, 2229(2010).
[40] C Zhang, Y Li, W Wang et al. A novel two-nozzle electrospinning process for preparing microfiber reinforced pH-sensitive nano-membrane with enhanced mechanical property. Eur Polym J, 47, 2228(2011).
[41] V C Diculescu, M Beregoi, A Evanghelidis et al. Palladium/palladium oxide coated electrospun fibers for wearable sweat pH-sensors. Sci Rep, 9, 8902(2019).
[42] C K da Silva, D J da S Mastrantonio, J A V Costa et al. Innovative pH sensors developed from ultrafine fibers containing açaí (Euterpe oleracea) extract. Food Chem, 294, 397(2019).
[43] M Gorji, A Sadeghianmaryan, H Rajabinejad et al. Development of highly pH-sensitive hybrid membranes by simultaneous electrospinning of amphiphilic nanofibers reinforced with graphene oxide. J Funct Biomater, 10, 23(2019).
[44] A Agarwal, A Raheja, T S Natarajan et al. Development of universal pH sensing electrospun nanofibers. Sens Actuators B, 161, 1097(2012).
[45] K Devarayan, B S Kim. Reversible and universal pH sensing cellulose nanofibers for health monitor. Sens Actuators B, 209, 281(2015).
[46] E Schoolaert, m R Hoogenboom, k K D Clerck. Colorimetric nanofibers as optical sensors. Adv Funct Mater, 27, 1702646(2017).
[47]
[48] E Schoolaert, I Steyaert, G Vancoillie et al. Blend electrospinning of dye-functionalized chitosan and poly(ε-caprolactone): towards biocompatible pH-sensors. J Mater Chem B, 4, 4507(2016).
[49] N Pan, J Qin, P Feng et al. Color-changing smart fibrous materials for naked eye real-time monitoring of wound pH. J Mater Chem B, 7, 2626(2019).
[50] X Yan, M H You, T Lou et al. Colorful hydrophobic poly(vinyl butyral)/cationic dye fibrous membranes via a colored solution electrospinning process. Nanoscale Res Lett, 11, 540(2016).
[51] M You, X Yan, J Zhang et al. Colorimetric humidity sensors based on electrospun polyamide/CoCl2 nanofibrous membranes. Nanoscale Res Lett, 12, 360(2017).
[52] M N Liu, X Yan, M H You et al. Reversible photochromic nanofibrous membranes with excellent water/windproof and breathable performance. J Appl Polym Sci, 135, 46342(2018).
[53] C Li, T Lou, X Yan et al. Fabrication of pure chitosan nanofibrous membranes as effective absorbent for dye removal. Int J Biol Macromol, 106, 768(2018).
[54] G S Liu, X Yan, F F Yan et al. In situ electrospinning iodine-based fibrous meshes for antibacterial wound dressing. Nanoscale Res Lett, 13, 309(2018).
[55] S Chen, G S Liu, H W He et al. Physical structure induced hydrophobicity analyzed from electrospinning and coating polyvinyl butyral films. Adv Cond Matter Phys, 2019, 6179456(2019).
[56] X Yan, M Yu, S Ramakrishna et al. Advances in portable electrospinning devices for in-situ delivery of personalized wound care. Nanoscale(2019).
[57] K I Priyadarsini. Photophysics, photochemistry and photobiology of curcumin: Studies from organic solutions, bio-mimetics and living cells. J Photochem Photobiol C, 10, 81(2009).
[58] D J Graham, B Jaselskis, C E Moore. Development of the glass electrode and the pH response. J Chem Educ, 90, 345(2013).
[59] T Guinovart, G V Ramírez, J R Windmiller et al. Bandage-based wearable potentiometric sensor for monitoring wound pH. Electroanalysis, 26, 1345(2014).
[60] W S Huang, B D Humphrey, A G MacDiarmid. Polyaniline, a novel conducting polymer. Morphology and chemistry of its oxidation and reduction in aqueous electrolytes. J Chem Soc Faraday Trans, 82, 2385(1986).
[61] S Quillard, K Berrada, G Louarn et al. In situ Raman spectroscopic studies of the electrochemical behavior of polyaniline. New J Chem, 19, 365(1995).
[62] T Lindfors, A Ivaska. pH sensitivity of polyaniline and its substituted derivatives. J Electroanal Chem, 531, 43(2002).
[63] M Kaempgen, S Roth. Transparent and flexible carbon nanotube/polyaniline pH sensors. J Electroanal Chem, 586, 72(2006).
[64] T Lindfors, S Ervela, A Ivaska. Polyaniline as pH-sensitive component in plasticized PVC membranes. J Electroanal Chem, 560, 69(2003).
[65] X Zhang, B Ogorevc, J Wang. Solid-state pH nanoelectrode based on polyaniline thin film electrodeposited onto ion-beam etched carbon fiber. Anal Chim Acta, 452, 1(2002).
[66] R Rahimi, M Ochoa, T Parupudi et al. A low-cost flexible pH sensor array for wound assessment. Sens Actuators B, 229, 609(2016).
[67] K J Loh, J P Lynch, N A Kotov. Inductively coupled nanocomposite wireless strain and pH sensors. Smart Struct Syst, 4, 531(2008).
[68] V Sridhar, K Takahata. A hydrogel-based passive wireless sensor using a flex-circuit inductive transducer. Sens Actuators A, 155, 58(2009).
[69] A Pal, D Goswami, H E Cuellar et al. Early detection and monitoring of chronic wounds using low-cost, omniphobic paper-based smart bandages. Biosens Bioelectron, 117, 696(2018).
[70] R Rahimi, U Brener, S Chittiboyina et al. Laser-enabled fabrication of flexible and transparent pH sensor with near-field communication for in-situ monitoring of wound infection. Sens Actuators B, 267, 198(2018).
[71] T Hizawa, K Sawada, H Takao et al. Fabrication of a two-dimensional pH image sensor using a charge transfer technique. Sens Actuators B, 117, 509(2006).
[72] M Punjiya, H Rezaei, M A Zeeshan et al. A flexible pH sensing smart bandage with wireless cmos readout for chronic wound monitoring. 19th International Conference on Solid-State Sensors Actuators and Microsystems (TRANSDUCERS), 1700(2017).
[73] B Lyu, M Punjiya, Z Matharu et al. An improved pH mapping bandage with thread-based sensors for chronic wound monitoring. IEEE International Symposium on Circuits and Systems (ISCAS), 1(2018).
[74] D C Christodouleas, B Kaur, P Chorti. From point-of-care testing to ehealth diagnostic devices (eDiagnostics). ACS Cent Sci, 4, 1600(2018).
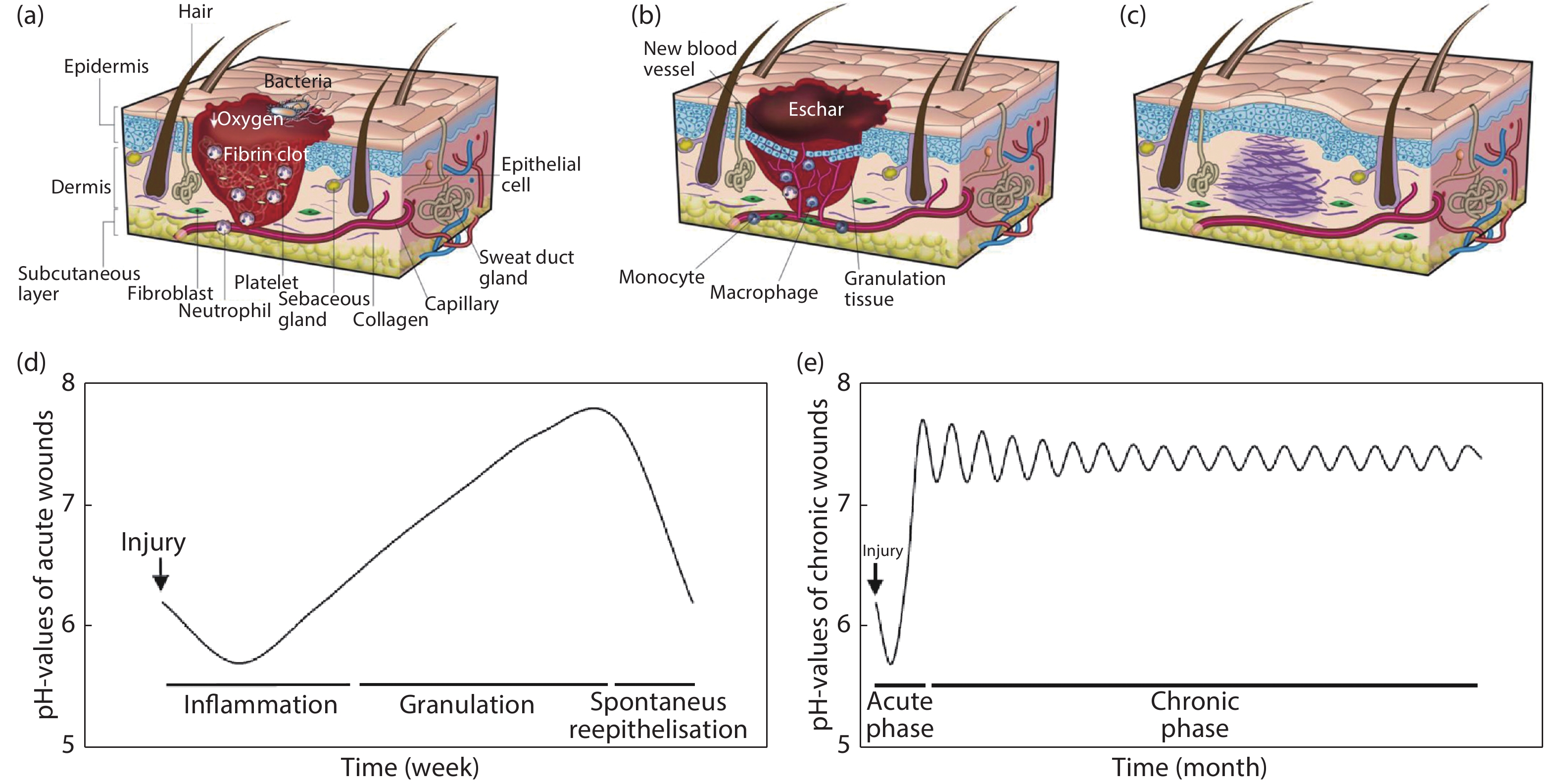
Set citation alerts for the article
Please enter your email address