
- Photonics Research
- Vol. 10, Issue 3, 628 (2022)
Abstract
1. INTRODUCTION
Owing to its outstanding optoelectronic properties, including flexible tunability of emission wavelength, long charge diffusion, high photoluminescence quantum yield (PLQY), large absorption coefficients, and narrow emission spectrum, all-inorganic halide perovskites nanomaterials
Nevertheless, the sensitivity and instability of perovskite to moisture and heat seriously affect the performance of the perovskite lasers. To address these issues, diverse strategies such as coating, surface ligand engineering, and doping/alloying heterogeneous atoms have been implemented [24–28]. For example, our group devised an effective methodology to fabricate perovskite core/shell QDs by capping
In view of previous studies, the weak binding energy between long-chain organic carbon chain ligands and QDs will lead to the aggregation of QDs and poor long-term stability, which has a great impact on the perovskite laser’s performance [16,36–40]. A facile ligand-engineering strategy was proposed to promote properties of
Sign up for Photonics Research TOC. Get the latest issue of Photonics Research delivered right to you!Sign up now
2. EXPERIMENT
The QDs solution was dropped to the glass (
The fundamental pulse at 800 nm from a Ti:sapphire laser (repetition rate: 1 kHz, pulse-width: 35 fs, Solstice, Spectra-Physics) was used as a pump source. All the lasing experiments were carried out at room temperature.
3. RESULTS AND DISCUSSION
Traditional hot injection was adopted to synthesize
Figure 1.(a) Passivation and ligand modification procedure on the surface of the
Figure 2.(a) XPS profiles for
Figure 3.(a) PL spectrum, (b) absorption spectrum, and (c) time-resolved PL decay of
As shown in Fig. 3(d), the PL intensity of
Previously, all-inorganic
Figure 4.(a) TEM image, (b) HRTEM image, and (c) element mapping of
To study the lasing properties, the well-dispersed
Figure 5.Random lasing from the composite film under two-photon excitation. (a) Schematic of random lasing from
Figure 6.Gaussian fitting of a selected lasing peak corresponding to the
4. CONCLUSION
In summary, a facile and valid ligand modification strategy is proposed to synthesize
References
[1] X. Li, Y. Wu, S. Zhang, B. Cai, Y. Gu, J. Song, H. Zeng. CsPbX3 quantum dots for lighting and displays: room-temperature synthesis, photoluminescence superiorities, underlying origins and white light emitting diodes. Adv. Funct. Mater., 26, 2435-2445(2016).
[2] L. Protesescu, S. Yakunin, M. I. Bodnarchuk, F. Krieg, R. Caputo, C. H. Hendon, R. X. Yang, A. Walsh, M. V. Kovalenko. Nanocrystals of cesium lead halide perovskites (CsPbX3, X = Cl, Br, and I): novel optoelectronic materials showing bright emission with wide color gamut. Nano Lett., 15, 3692-3696(2015).
[3] J. Song, J. Li, X. Li, L. Xu, Y. Dong, H. Zeng. Nanocrystals: quantum dot light-emitting diodes based on inorganic perovskite cesium lead halides (CsPbX3). Adv. Mater., 27, 7162-7167(2015).
[4] X. Du, G. Wu, J. Cheng, H. Dang, K. Ma, Y.-W. Zhang, P.-F. Tan, S. Chen. High quality CsPbBr3 perovskite nanocrystals for quantum dot light-emitting diodes. RSC Adv., 7, 10391-10396(2017).
[5] J. Li, L. Xu, T. Wang, J. Song, J. Chen, J. Xue, Y. Dong, B. Cai, Q. Shan, B. Han, H. Zeng. 50-fold EQE improvement up to 6.27% of solution-processed all-inorganic perovskite CsPbBr3 QLEDs via surface ligand density control. Adv. Mater., 29, 1603885(2017).
[6] A. Swarnkar, A. R. Marshall, E. M. Sanehira, B. D. Chernomordik, D. T. Moore, J. A. Christians, T. Chakrabarti, J. M. Luther. Quantum dot–induced phase stabilization of α-CsPbI3 perovskite for high-efficiency photovoltaics. Science, 354, 92-95(2016).
[7] M. Yuan, L. N. Quan, R. Comin, G. Walters, R. Sabatini, O. Voznyy, S. Hoogland, Y. Zhao, E. M. Beauregard, P. Kanjanaboos, Z. Lu, D. H. Kim, E. H. Sargent. Perovskite energy funnels for efficient light-emitting diodes. Nat. Nanotechnol., 11, 872-877(2016).
[8] Q. Chen, J. Wu, X. Ou, B. Huang, J. Almutlaq, A. A. Zhumekenov, X. Guan, S. Han, L. Liang, Z. Yi, J. Li, X. Xie, Y. Wang, Y. Li, D. Fan, D. B. L. Teh, A. H. All, O. F. Mohammed, O. M. Bakr, T. Wu, M. Bettinelli, H. Yang, W. Huang, X. Liu. All-inorganic perovskite nanocrystal scintillators. Nature, 561, 88-93(2018).
[9] Y. Chen, Y. Chu, X. Wu, W. Ou-Yang, J. Huang. High-performance inorganic perovskite quantum dot-organic semiconductor hybrid phototransistors. Adv. Mater., 29, 1704062(2017).
[10] X. Song, X. Liu, D. Yu, C. Huo, J. Ji, X. Li, S. Zhang, Y. Zou, G. Zhu, Y. Wang, M. Wu, A. Xie, H. Zeng. Boosting two-dimensional MoS2/CsPbBr3 photodetectors via enhanced light absorbance and interfacial carrier separation. ACS Appl. Mater. Interfaces, 10, 2801-2809(2018).
[11] Y. Dong, Y. Zou, J. Song, X. Song, H. Zeng. Recent progress of metal halide perovskite photodetectors. J. Mater. Chem. C, 5, 11369-11394(2017).
[12] Z. Yong, Y. Zhou, J. Ma, Y. Chen, J. Yang, Y. Song, J. Wang, H. Sun. Controlling crystallization of all-inorganic perovskite films for ultralow-threshold amplification spontaneous emission. ACS Appl. Mater. Interfaces, 9, 32920-32929(2017).
[13] Y. Wang, X. Li, X. Zhao, L. Xiao, H. Zeng, H. Sun. Nonlinear absorption and low-threshold multiphoton pumped stimulated emission from all-inorganic perovskite nanocrystals. Nano Lett., 16, 448-453(2016).
[14] Z. Hu, Z. Liu, Z. Zhan, T. Shi, J. Du, X. Tang, Y. Leng. Advances in metal halide perovskite lasers: synthetic strategies, morphology control, and lasing emission. Adv. Photon., 3, 034002(2021).
[15] Y. Xu, Q. Chen, C. Zhang, R. Wang, H. Wu, X. Zhang, G. Xing, W. W. Yu, X. Wang, Y. Zhang, M. Xiao. Two-photon-pumped perovskite semiconductor nanocrystal lasers. J. Am. Chem. Soc., 138, 3761-3768(2016).
[16] S. W. Eaton, M. Lai, N. A. Gibson, A. B. Wong, L. Dou, J. Ma, L.-W. Wang, S. R. Leone, P. Yang. Lasing in robust cesium lead halide perovskite nanowires. Proc. Natl. Acad. Sci. USA, 113, 1993-1998(2016).
[17] Z. Liu, M. Hu, J. Du, T. Shi, Z. Wang, Z. Zhang, Z. Hu, Z. Zhan, K. Chen, W. Liu, J. Tang, H. Zhang, Y. Leng, R. Li. Subwavelength-polarized quasi-two-dimensional perovskite single-mode nanolaser. ACS Nano, 15, 6900-6908(2021).
[18] S. Yakunin, L. Protesescu, F. Krieg, M. I. Bodnarchuk, G. Nedelcu, M. Humer, G. De Luca, M. Fiebig, W. Heiss, M. V. Kovalenko. Low threshold amplified spontaneous emission and lasing from colloidal nanocrystals of cesium lead halide perovskites. Nat. Commun., 6, 8056(2015).
[19] J. Pan, S. P. Sarmah, B. Murali, I. Dursun, W. Peng, M. R. Parida, J. Liu, L. Sinatra, N. Alyami, C. Zhao, E. Alarousu, T. K. Ng, B. S. Ooi, O. M. Bakr, O. F. Mohammed. Air-stable surface-passivated perovskite quantum dots for ultra-robust, single- and two-photon-induced amplified spontaneous emission. J. Phys. Chem. Lett., 6, 5027-5033(2015).
[20] X. Chen, K. Wang, B. Shi, T. Liu, R. Chen, M. Zhang, W. Wen, G. Xing, J. Wu. All-inorganic perovskite nanorod arrays with spatially randomly distributed lasing modes for all-photonic cryptographic primitives. ACS Appl. Mater. Interfaces, 13, 30891-30901(2021).
[21] Y. Lu, T. L. Shen, K. Peng, P. Cheng, S. Chang, M. Lu, C. W. Chu, T. Guo, H. A. Atwater. Up conversion plasmonic lasing from an organolead trihalide perovskite nanocrystal with low threshold. ACS Photon., 8, 335-342(2021).
[22] Q. Xiong, S. Huang, J. Du, X. Tang, F. Zeng, Z. Liu, Z. Zhang, T. Shi, J. Yang, D. Wu, H. Lin, Z. Luo, Y. Leng. Surface ligand engineering for CsPbBr3 quantum dots aiming at aggregation suppression and amplified spontaneous emission improvement. Adv. Opt. Mater., 8, 2000977(2020).
[23] Y. Wang, M. Zhi, Y. Chang, J. Zhang, Y. Chan. Stable, ultralow threshold amplified spontaneous emission from CsPbBr3 nanoparticles exhibiting trion gain. Nano Lett., 18, 4976-4984(2018).
[24] Z.-J. Li, E. Hofman, J. Li, A. H. Davis, C.-H. Tung, L.-Z. Wu, W. Zheng. Photoelectrochemically active and environmentally stable CsPbBr3/TiO2 core/shell nanocrystals. Adv. Funct. Mater., 28, 1704288(2018).
[25] S. Wang, J. Yu, M. Zhang, D. Chen, C. Li, R. Chen, G. Jia, A. L. Rogach, X. Yang. Stable, strongly emitting cesium lead bromide perovskite nanorods with high optical gain enabled by an intermediate monomer reservoir synthetic strategy. Nano Lett., 19, 6315-6322(2019).
[26] H. C. Wang, S. Y. Lin, A. C. Tang, B. P. Singh, H. C. Tong, C. Y. Chen, Y. C. Lee, T. L. Tsai, R. S. Liu. “Mesoporous silica particles integrated with all-inorganic CsPbBr3 perovskite quantum-dot nanocomposites (MP-PQDs) with high stability and wide color gamut used for backlight display. Angew. Chem., 55, 7924-7929(2016).
[27] X. Liu, X. Zhang, L. Li, J. Xu, S. Yu, X. Gong, J. Zhang, H. Yin. Stable luminescence of CsPbBr3/nCdS core/shell perovskite quantum dots with Al self-passivation layer modification. ACS Appl. Mater. Interfaces, 11, 40923-40931(2019).
[28] T. Xuan, X. Yang, S. Lou, J. Huang, Y. Liu, J. Yu, H. Li, K. L. Wong, C. Wang, J. Wang. Highly stable CsPbBr3 quantum dots coated with alkyl phosphate for white light-emitting diodes. Nanoscale, 9, 15286-15290(2017).
[29] X. Tang, J. Yang, S. Li, Z. Liu, Z. Hu, J. Hao, J. Du, Y. Leng, H. Qin, X. Lin, Y. Lin, Y. Tian, M. Zhou, Q. Xiong. Single halide perovskite/semiconductor core/shell quantum dots with ultrastability and nonblinking properties. Adv. Sci., 6, 1900412(2019).
[30] W. J. Mir, Y. Mahor, A. Lohar, M. Jagadeeswararao, S. Das, S. Mahamuni, A. Nag. Postsynthesis doping of Mn and Yb into CsPbX3 (X = Cl, Br, or I) perovskite nanocrystals for down-conversion emission. Chem. Mater., 30, 8170-8178(2018).
[31] F. Krieg, S. T. Ochsenbein, S. Yakunin, S. ten Brinck, P. Aellen, A. Sness, B. Clerc, D. Guggisberg, O. Nazarenko, Y. Shynkarenko, S. Kumar, C. J. Shih, I. Infante, M. V. Kovalenko. Colloidal CsPbX3 (X = Cl, Br, I) nanocrystals 2.0: Zwitterionic capping ligands for improved durability and stability. ACS Energy Lett., 3, 641-646(2018).
[32] V. K. Ravi, P. K. Santra, N. Joshi, J. Chugh, S. K. Singh, H. Rensmo, P. Ghosh, A. Nag. Origin of the substitution mechanism for the binding of organic ligand on the surface of CsPbBr3 perovskite nanocubes. J. Phys. Chem. Lett., 8, 4988-4994(2017).
[33] C. Dong, Y. Xiao, Z. Han, G. Guo, X. Jiang, L. Tong, C. Gu, H. Ming. Low-threshold microlaser in Er:Yb phosphate glass coated microsphere. IEEE Photon. Technol. Lett., 20, 342-344(2008).
[34] X. Li, F. Cao, D. Yu, J. Chen, Z. Sun, Y. Shen, Y. Zhu, L. Wang, Y. Wei, Y. Wu, H. Zeng. All inorganic halide perovskites nanosystem: synthesis, structural features, optical properties and optoelectronic applications. Small, 13, 1603996(2017).
[35] A. Pan, B. He, X. Fan, Z. Liu, J. J. Urban, A. P. Alivisatos, L. He, Y. Liu. Insight into the ligand-mediated synthesis of colloidal CsPbBr3 perovskite nanocrystals: the role of organic acid, base, and cesium precursors. ACS Nano, 10, 7943-7954(2016).
[36] M. L. De Giorgi, F. Krieg, M. V. Kovalenko, M. Anni. Amplified spontaneous emission threshold reduction and operational stability improvement in CsPbBr3 nanocrystals films by hydrophobic functionalization of the substrate. Sci. Rep., 9, 17964(2019).
[37] K. Ren, J. Wang, S. Chen, Q. Yang, J. Tian, H. Yu, M. Sun, X. Zhu, S. Yue, Y. Sun, K. Liu, M. Azam, Z. Wang, P. Jin, S. Qu, Z. Wang. Realization of perovskite-nanowire-based plasmonic lasers capable of mode modulation. Laser Photon. Rev., 13, 1800306(2019).
[38] Y.-F. Xiao, B.-B. Li, X. Jiang, X. Hu, Y. Li, Q. Gong. High quality factor, small mode volume, ring-type plasmonic micro resonator on a silver chip. J. Phys. B, 43, 035402(2010).
[39] H. Zhu, Y. Fu, F. Meng, X. Wu, Z. Gong, Q. Ding, M. V. Gustafsson, M. T. Trinh, S. Jin, X. Y. Zhu. Lead halide perovskite nanowire lasers with low lasing thresholds and high quality factors. Nat. Mater., 14, 636-642(2015).
[40] D. Yan, T. Shi, Z. Zang, T. Zhou, Z. Liu, Z. Zhang, J. Du, Y. Leng, X. Tang. Ultrastable CsPbBr3 perovskite quantum dot and their enhanced amplified spontaneous emission by surface ligand modification. Small, 15, 1901173(2019).
[41] W. Yang, L. Fei, F. Gao, W. Liu, H. Xu, L. Yang, Y. Liu. Thermal polymerization synthesis of CsPbBr3 perovskite-quantum-dots@copolymer composite: towards long-term stability and optical phosphor application. Chem. Eng. J., 387, 124180(2020).
[42] A. Mikosch, S. Ciftci, G. Tainter, R. Shivanna, B. Haehnle, F. Deschler, A. J. C. Kuehne. Laser emission from self-assembled colloidal crystals of conjugated polymer particles in a metal-halide perovskite matrix. Chem. Mater., 31, 2590-2596(2019).
[43] H. Zhang, L. Yuan, Y. Chen, Y. Zhang, Y. Yu, X. Liang, W. Xiang, T. Wang. Amplified spontaneous emission and random lasing using CsPbBr3 quantum dot glass through controlling crystallization. Chem. Commun., 56, 2853-2856(2020).
[44] S. Yuan, D. Chen, X. Li, J. Zhong, X. Xu.
[45] S. Liu, G. Shao, L. Ding, J. Liu, W. Xiang, X. Liang. Sn-doped CsPbBr3 QDs glass with excellent stability and optical properties for WLED. Chem. Eng. J., 361, 937-944(2019).
[46] S. Aharon, M. Wierzbowska, L. Etgar. The effect of the alkylammonium ligand’s length on organic–inorganic perovskite nanoparticles. ACS Energy Lett., 3, 1387-1393(2018).
[47] M. C. Brennan, J. E. Herr, T. S. Nguyen-Beck, J. Zinna, S. Draguta, S. Rouvimov, J. Parkhill, M. Kuno. Origin of the size-dependent Stokes shift in CsPbBr3 perovskite nanocrystals. J. Am. Chem. Soc., 139, 12201-12208(2017).
[48] A. Chiasera, Y. Dumeige, P. Féron, M. Ferrari, Y. Jestin, G. N. Conti, S. Pelli, S. Soria, G. C. Righini. Spherical whispering-gallery-mode microresonators. Laser Photon. Rev., 4, 457-482(2010).
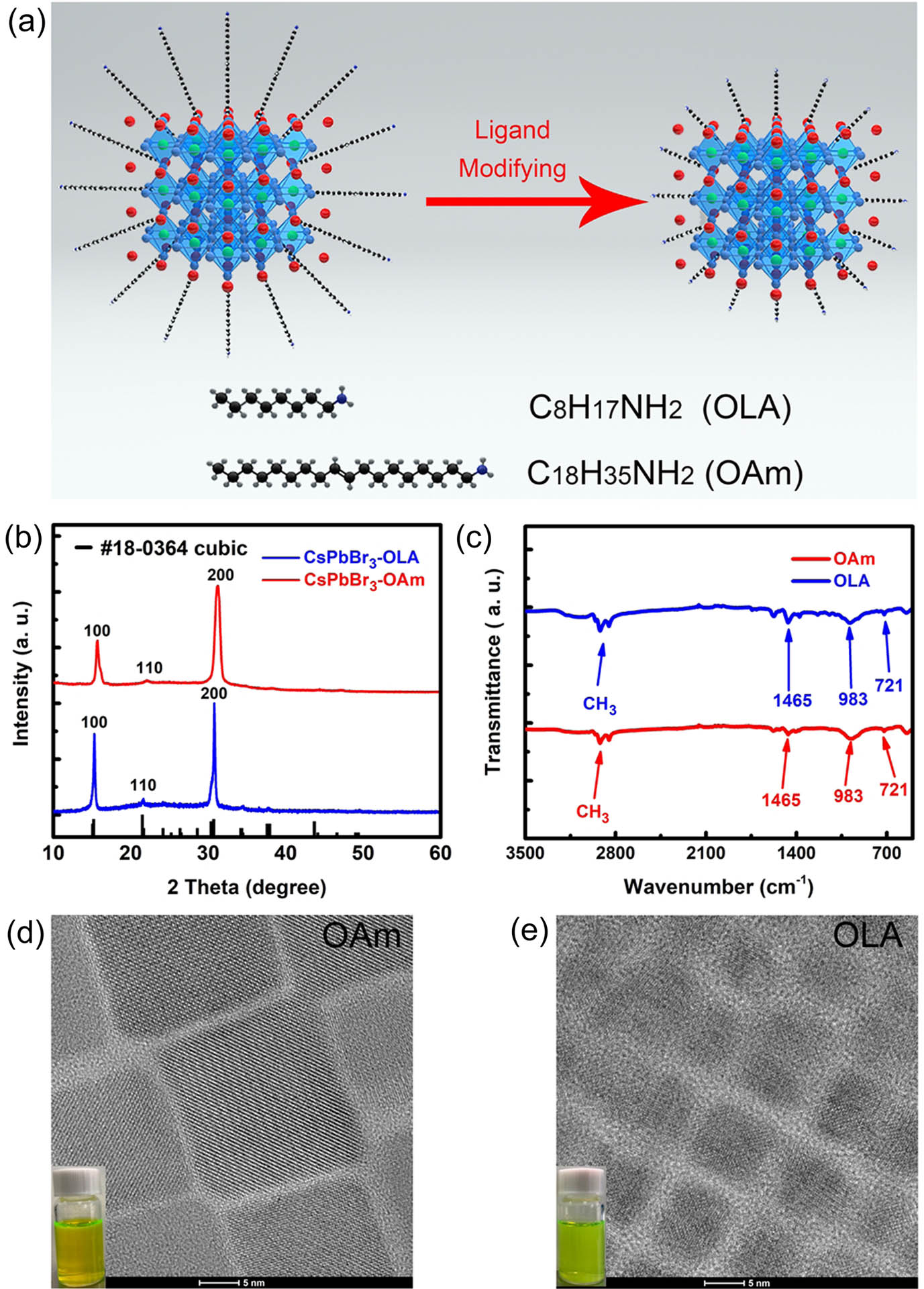
Set citation alerts for the article
Please enter your email address