Author Affiliations
1School of Physical Sciences, University of Science and Technology of China, Hefei 230026, China2Key Laboratory of High Power Laser Materials, Shanghai Institute of Optics and Fine Mechanics, Chinese Academy of Sciences, Shanghai 201800, China3Accelink Technologies Co., Wuhan 430000, China4Hangzhou Institute for Advanced Study, University of Chinese Academy of Sciences, Hangzhou 310024, China5College of Materials Science and Opto-Electronic Technology, University of Chinese Academy of Sciences, Beijing 100049, Chinashow less
Abstract
In this Letter, the optical amplification characteristics of the home-made Bi/P co-doped silica fiber were systematically explored in the range of 1270–1360 nm. The maximum gain of 24.6 dB was obtained in the single-pass amplification device, and then improved to 38.3 dB in the double-pass amplification device for signal power. In addition, we simultaneously investigated the laser performance of the fiber with the linear cavity. A slope efficiency of 16.4% at was obtained with a maximum output power of about 133 mW under the input pump power of 869 mW at 1240 nm. As far as we know, it is the first laser reported based on the bismuth-doped fiber in China.1. Introduction
For the past few years, bismuth-doped fibers have been one of the most promising materials for developing amplifiers and lasers outside conventional C+L band due to its ultra-wide near-infrared emission range[1,2]. In 2005, Dvoyrin et al. successfully developed the first bismuth-doped fiber by using the modified chemical vapor deposition (MCVD) technique[3]. Soon afterward, they achieved a continuous wavelength (CW) laser in the range of 1150–1300 nm[4]. Since then, more in-depth research has been carried out on bismuth-doped fibers[5–11]. As generally acknowledged, the co-doped elements and valence states of bismuth ions are the key factors in determining the performance of the fiber[12].
So far, the bismuth-doped fiber amplifiers (BDFAs) and lasers (BDFLs) based on the bismuth-doped phosphosilicate fibers operating in the 1300 nm band have been reported abroad[2,13–21], which have crucial applications in biological imaging, medical treatment, and optical fiber communications[22–25]. In 2019, Thipparapu et al. demonstrated an all-fiber laser at 1340 nm by a 70 m bismuth-doped phosphosilicate fiber, the efficiency of which was 14.5% with the output power over 100 mW[2]. Further, a tunable BDFL operating in the 1320–1370 nm range was constructed by using a 200 m bismuth-doped phosphosilicate fiber. The output power over 100 mW with a slope efficiency of more than 25% was obtained over the whole 50 nm tuning band under pumping of two 1270 nm laser diodes (LDs)[26]. In addition, the optical amplification characteristics of the single-pass BDFA were compared with those of the double-pass configuration with a 150 m fiber. The gain and noise figure (NF) of the signal-pass amplifier were and , respectively, with the input signal power of in the range of 1325 to 1360 nm, whereas the maximum gain of the double-pass amplifier was improved to 40 dB at 1360 nm for the input signal power of [27]. Recently, we have successfully fabricated a Bi/P co-doped silica fiber (BPSF) with low loss and realized the optical amplification in the range of 1355–1385 nm[28]. Then, the core absorption coefficient of the fiber was improved by optimizing the preparation process, and a maximum gain of the BDFA was increased to about 20 dB at 1355 nm[29]. However, the gap about the gain coefficient of the bismuth-doped fiber between China and foreign countries is still wide, and there are currently no reports on BDFLs at home.
In this paper, a BPSF with improved performance was fabricated and used to build amplifiers operating in the O-band. A maximum gain of 24.6 dB was measured in the single-pass configuration, whereas that was improved to 38.3 dB in the double-pass setup for signal power of at 1330 nm. The maximum gain was higher than that reported previously by us. Additionally, we first demonstrated an all-fiber laser based on the home-made BPSF; the maximum output power and the slope efficiency were and at 1313 nm, respectively.
Sign up for Chinese Optics Letters TOC. Get the latest issue of Chinese Optics Letters delivered right to you!Sign up now
2. Experiment and Methods
The BPSF was prepared by the traditional MCVD technique with the same fiber parameters as previously reported[29]. The core absorption spectrum was evaluated by the conventional cut-back method. The relation between the absorption coefficient and the pump power was measured with a short length of BPSF at 1240 nm[30].
Figure 1 depicts the experimental device to compare the amplification performance of the single- (solid, without circulators) and double-pass (dashed) BDFA. The pump sources were two 1240 nm LDs with the maximum output power of , protected by optical isolators (ISOs). The signal light was provided by a tunable laser source (TLS) in the range of 1260–1360 nm and the power was attenuated by the attenuator (ATT). The pump and signal light were combined and separated through wavelength division multiplexers (WDMs). The double-pass configuration was constructed by connecting port 2 and port 3 of circulator 2 (C2) to the couple signal into the fiber again, and the amplified signal was recorded in port 3 of circulator 1 (C1).
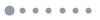
Figure 1.Experimental setup of the single-pass (solid, without C1 and C2) and double-pass (dashed) BDFA.
The experimental scheme of the BDFL is shown in Fig. 2. A pair of fiber Bragg gratings (FBGs) was used to form the laser cavity. The center wavelength and reflectivity of the high reflectivity FBG (HR FBG) in the laser cavity were 1313.63 nm and above 99%, respectively. Three FBGs with reflectivities of 10.4%, 30.8%, and 51% were used as the output coupler FBGs (OC FBGs). The 3 dB bandwidths of the FBGs were , 0.03 nm, 0.05 nm, and 0.06 nm, respectively. The output spectrum and power of the laser were measured by an optical spectrum analyzer (OSA) and a power meter.
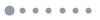
Figure 2.Experimental setup of the BDFL.
3. Result and Discussion
Figure 3(a) illustrates the core absorption spectrum of the BPSF, and the cross section of the fiber is shown in the inset. The absorption coefficient was improved to at 1240 nm. It was as low as 20 dB/km at a wavelength longer than 1500 nm. Figure 3(b) shows the relation between the loss and the pump power at 1240 nm, and there was an unsaturable loss (UL) for the high pump power, the percentage of which in the small signal absorption was about 28.3%.
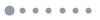
Figure 3.(a) Absorption spectrum (the inset shows the cross section) and (b) unsaturable loss of the BPSF.
Figure 4 depicts the optical amplification characteristics of the single-pass amplifier with a 200 m BPSF. A 3 dB bandwidth reached about 45 nm. A gain of 24.6 dB was maximum, and an NF was 4.8 dB at 1330 nm under the total pump power of 785 mW. Moreover, Fig. 4(b) shows the amplifier character under different pump powers as the input signal power was at 1330 nm. When the pump power was improved from 428 to 785 mW, an increase of gain was about 3 dB with a coefficient of 0.051 dB/mW, and the NF decreased by 0.3 dB. Moreover, the gain and NF as functions of the signal power at 1330 nm are shown in Fig. 4(c). When the signal power was improved from to 0 dBm with the fixed input pump one of 785 mW, the gain decreased from 24.6 to 15.5 dB due to gain saturation, and the NF increased to 6.2 dB.
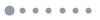
Figure 4.The optical amplification characteristics of the single-pass fiber amplifier for a 200 m BPSF.
The amplifier performance in the double-pass setup under the same conditions was also evaluated, as shown in Fig. 5. Compared with that in the single-pass setup, the maximum gain increased to 38.3 dB with an improvement of 55%, and an NF increased from 4.8 dB to 6.4 dB at 1330 nm. The gain of the double-pass amplifier reached above 27 dB in the range of 1300–1360 nm.
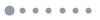
Figure 5.The amplification characteristics of the 200 m BPSF in the single-pass and double-pass amplifiers with the signal power of −30 dBm and the pump one of 785 mW at 1240 nm.
The amplification characteristics of the double-pass amplifier as functions of the pump or the signal power are shown in Fig. 6. As the pump power increased, the gain and NF showed the same trends as those in the single pass. Moreover, the gain coefficient, with respect to the pump power, was improved to 0.062 dB/mW by 1.22%. At the same time, the variations of the gain and the NF under different signal powers are shown in Fig. 6(b). The gain decreased to 17.9 dB due to gain saturation, and the NF increased to 9.1 dB at the input signal power of 0 dBm.
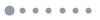
Figure 6.The variations of the gain and the NF under different (a) pump and (b) signal powers in the double-pass amplification setup.
The measured gain spectrum of the BPSF suggested the possibility of designing a fiber laser in the O-band [see Fig. 3(a)]. Due to the limitations of the FBG, the laser performance of the fiber with a linear cavity scheme was evaluated only at . The slope efficiency of the laser as a function of the fiber length for different reflectivity of the OC FBGs is illustrated in Fig. 7(a). Lowering the reflectivity of the OC FBG resulted in an increment of the slope efficiency. A maximum value of 16.4% was achieved for a fiber length of 96 m and a reflectivity of 10.4%. The relation between the output power and the pump power is shown in Fig. 7(b). The output spectrum of the laser resolution bandwidth of 0.02 nm measured with an OSA is shown in the inset. The maximum output power was about 133 mW. The linewidth and the optical signal-to-noise ratio (OSNR) of the output laser observed at 1313.6 nm were and more than 50 dB, respectively. The laser behaviors of our BDFL were limited by a low concentration of efficient Bi ions and high unsaturable optical loss in the fiber. In the future, the fiber fabrication process will be further optimized to decrease the unsaturable optical loss and improve the efficiency of the BDFAs and the BDFLs.
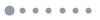
Figure 7.(a) The dependence of the laser slope efficiency on the fiber length for different reflectivities of the OC FBGs. (b) The relation between the output power and the pump power (the inset shows the laser spectrum measured at ∼1313 nm).
4. Conclusion
In this paper, the optical amplification and laser characteristics of a BPSF fabricated by the MCVD method were studied. We systematically studied the amplification characteristics of the single-pass and double-pass fiber amplifier in the O-band. The highest gain of about 38 dB and an NF of 6.4 dB were obtained in the double-pass amplification configuration at 1330 nm. Additionally, a BDFL with the output power of about 133 mW and a slope efficiency of 16.4% at was demonstrated. To the best of our knowledge, this is the first time achieving laser output by using a home-made BPSF. This work lays the foundation for subsequent research on bismuth-doped fiber amplifiers and lasers in China.
References
[1] J. K. Sahu, N. K. Thipparapu, A. A. Umnikov, P. Barua. Recent advances in Bi-doped fiber lasers and amplifiers. 11th International Symposium on SiO2, Advanced dielectrics and Related Devices(2016).
[2] N. K. Thipparapu, Y. Wang, S. Wang, A. A. Umnikov, P. Barua, J. K. Sahu. Bi-doped fiber amplifiers and lasers [Invited]. Opt. Mater. Express, 9, 2446(2019).
[3] V. V. Dvoyrin, V. M. Mashinsky, E. M. Dianov, A. A. Umnikov, M. V. Yashkov, A. N. Guryanov. Absorption, fluorescence and optical amplification in MCVD bismuth-doped silica glass optical fibres. 31st European Conference on Optical Communication(2005).
[4] E. M. Dianov, V. V. Dvoyrin, V. M. Mashinsky, A. A. Umnikov, M. V. Yashkov, A. N. Gur’yanov. CW bismuth fibre laser. Quantum Electron., 35, 1083(2005).
[5] B. Denker, B. Galagan, V. Osiko, S. Sverchkov, E. Dianov. Luminescent properties of Bi-doped boro-alumino-phosphate glasses. Appl. Phys. B, 87, 135(2007).
[6] S. Yoo, M. P. Kalita, J. Nilsson, J. Sahu. Excited state absorption measurement in the 900–1250 nm wavelength range for bismuth-doped silicate fibers. Opt. Lett., 34, 530(2009).
[7] Y. Fujimoto. Local structure of the infrared bismuth luminescent center in bismuth-doped silica glass. J. Am. Ceram. Soc., 93, 581(2010).
[8] M. Peng, G. Dong, L. Wondraczek, L. Zhang, N. Zhang, J. Qiu. Discussion on the origin of NIR emission from Bi-doped materials. J. Non-Cryst. Solids, 357, 2241(2011).
[9] J. Wen, T. Wang, F. Pang, X. Zeng, Z. Chen, G.-D. Peng. Photoluminescence characteristics of Bi(m+)-doped silica optical fiber: structural model and theoretical analysis. Jpn. J. Appl. Phys., 52, 122501(2013).
[10] D. A. Dvoretskii, I. A. Bufetov, V. V. Vel’miskin, A. S. Zlenko, V. F. Khopin, S. L. Semjonov, A. N. Guryanov, L. K. Denisov, E. M. Dianov. Optical properties of bismuth-doped silica fibres in the temperature range 300–1500 K. Quantum Electron., 42, 762(2012).
[11] P. Liu, L. Liao, Y. Chu, Y. Wang, X. Hu, J. Peng, J. Li, N. Dai. Irradiation and temperature influence on the Bi-doped silica fiber. Acta. Phys. Sin., 64, 224220(2015).
[12] E. M. Dianov. Bismuth-doped optical fibers: a challenging active medium for near-IR lasers and optical amplifiers. Light Sci. Appl., 1, e12(2012).
[13] V. V. Dvoyrin, O. I. Medvedkov, V. M. Mashinsky, A. A. Umnikov, A. N. Guryanov, E. M. Dianov. Optical amplification in 1430–1495 nm range and laser action in Bi-doped fibers. Opt. Express, 16, 16971(2008).
[14] E. M. Dianov, S. V. Firstov, V. F. Khopin, A. N. Gur’yanov, I. A. Bufetov. Bi-doped fibre lasers and amplifiers emitting in a spectral region of 1.3 µm. Quantum Electron., 38, 615(2008).
[15] E. M. Dianov, M. A. Mel’kumov, A. V. Shubin, S. V. Firstov, V. F. Khopin, A. N. Gur’yanov, I. A. Bufetov. Bismuth-doped fibre amplifier for the range 1300–1340 nm. Quantum Electron., 39, 1099(2009).
[16] M. A. Melkumov, I. A. Bufetov, A. V. Shubin, S. V. Firstov, V. F. Khopin, A. N. Guryanov, E. M. Dianov. Laser diode pumped bismuth-doped optical fiber amplifier for 1430 nm band. Opt. Lett., 36, 2408(2011).
[17] E. M. Dianov, A. V. Shubin, M. A. Melkumov, O. I. Medvedkov, I. A. Bufetov. High-power cw bismuth-fiber lasers. J. Opt. Soc. Am. B, 24, 1749(2007).
[18] V. M. Paramonov, M. I. Belovolov, V. F. Khopin, A. N. Gur’yanov, S. A. Vasil’ev, O. I. Medvedkov, M. A. Mel’kumov, E. M. Dianov. Bismuth-doped fibre laser continuously tunable within the range from 1.36 to 1.51 µm. Quantum Electron., 46, 1068(2016).
[19] M. A. Melkumov, V. Mikhailov, A. M. Hegai, K. E. Riumkin, P. S. Westbrook, D. J. DiGiovanni, E. M. Dianov. E-band data transmission over 80 km of non-zero dispersion fibre link using bismuth-doped fibre amplifier. Electron. Lett., 53, 1661(2017).
[20] V. M. Paramonov, S. A. Vasil’ev, O. I. Medvedkov, S. V. Firstov, M. A. Melkumov, V. F. Khopin, A. N. Gur’yanov, E. M. Dianov. Continuous-wave bismuth fibre laser tunable from 1.65 to 1.8 µm. Quantum Electron., 47, 1091(2017).
[21] S. V. Firstov, S. V. Alyshev, K. E. Riumkin, A. M. Khegai, A. V. Kharakhordin, M. A. Melkumov, E. M. Dianov. Laser-active fibers doped with bismuth for a wavelength region of 1.6–1.8 µm. IEEE J. Sel. Top. Quantum Electron., 24, 0902415(2018).
[22] C. Cleff, F. Ramos-Gomes, T. Bergmann, L. Bonacina, U. Weikert, M. Mitkovski, M. Schuette, F. Alves, M. Mei. 1300 nm fiber laser system for THG and 2PEF bio-imaging. Conference on Lasers and Electro-Optics(2016).
[23] Y.-J. You, C. Wang, Y.-L. Lin, A. Zaytsev, P. Xue, C.-L. Pan. Ultrahigh-resolution optical coherence tomography at 1.3 µm central wavelength by using a supercontinuum source pumped by noise-like pulses. Laser Phys. Lett., 13, 025101(2016).
[24] Y. Hong, K. R. H. Bottrill, Y. Wang, N. K. Thipparapu, J. K. Sahu, P. Petropoulos, D. J. Richardson. O+E-band transmission over 50-km SMF using a broadband bismuth doped fibre amplifier. Conference on Lasers and Electro-Optics Europe & European Quantum Electronics Conference (CLEO/Europe-EQEC), 1(2021).
[25] I. A. Bufetov, M. A. Melkumov, S. V. Firstov, K. E. Riumkin, A. V. Shubin, V. F. Khopin, A. N. Guryanov, E. M. Dianov. Bi-doped optical fibers and fiber lasers. IEEE J. Sel. Top. Quantum Electron., 20, 111(2014).
[26] S. Wang, Y. Wang, N. K. Thipparapu, M. Ibsen, D. J. Richardson, J. K. Sahu. Tunable CW Bi-doped fiber laser system from 1320 to 1370 nm using a fiber Bragg Grating. IEEE Photon. Technol. Lett., 32, 1443(2020).
[27] N. K. Thipparapu, Y. Wang, A. A. Umnikov, P. Barua, D. J. Richardson, J. K. Sahu. 40 dB gain all fiber bismuth-doped amplifier operating in the O-band. Opt. Lett., 44, 2248(2019).
[28] M. Guo, J. Tian, F. Wang, Q. Yang, C. Shao, M. Wang, L. Zhang, S. Cui, C. Yu, L. Hu. Amplification in E band based on highly phosphorus and bismuth co-doped silica fiber. Chinese J. Lumin., 43, 478(2022).
[29] J. Tian, M. Guo, F. Wang, C. Yu, L. Zhang, M. Wang, L. Hu. High gain E-band amplification based on the low loss Bi/P co-doped silica fiber. Chin. Opt. Lett., 20, 100602(2022).
[30] M. P. Kalita, S. Yoo, J. Sahu. Bismuth doped fiber laser and study of unsaturable loss and pump induced absorption in laser performance. Opt. Express, 16, 21032(2008).