Z. Y. Xu1,2, Y. H. Li1,3,*, and L. J. Wang1,3
Abstract
We demonstrated long-period grating (LPG) inscription on polymer functionalized optical microfibers and its applications in optical sensing. Optical microfibers were functionalized with ultraviolet-sensitive polymethyl methacrylate jackets and, thus, LPGs could be inscribed on optical microfibers via point-by-point ultraviolet laser exposure. For a 2 mm long microfiber LPG (MLPG) inscribed on optical microfibers with a diameter of 5.4 μm, a resonant dip of 15 dB at 1377 nm was observed. This MLPG showed a high sensitivity of strain and axial force, i.e., ?1.93 pm∕με and ?1.15 pm∕μN, respectively. Although the intrinsic temperature sensitivity of the LPGs is relatively low, i.e., ?12.75 pm∕°C, it can be increased to be ?385.11 pm∕°C by appropriate sealing. Benefiting from the small footprint and high sensitivity, MLPGs could have potential applications in optical sensing of strain, axial force, and temperature.1. INTRODUCTION
Optical microfibers have attracted much attention because of the high fractional evanescent field, wavelength/subwavelength diameters, low loss, easy fabrication, and full integration with fiber systems [1–3]. Numerous applications of optical microfibers, such as highly efficient light launching [4], miniature optical devices [5,6], and optical sensing [7,8], have been widely investigated. When functionalized with jackets and microstructures on the surface, optical microfibers could have more applications, such as sensitive optical sensors [9], optical amplifiers [10,11], and ultrafast all-optical modulators [12]. Among these applications, long-period gratings (LPGs) are among the most important and widely used components.
An LPG can be inscribed on optical microfibers, with the advantage of compactness due to the shorter grating pitches compared with LPGs in conventional optical fibers [13]. Moreover, the high fractional evanescent field in microfiber LPGs (MLPGs) promises possible applications in the sensing of surroundings, such as the refractive index of surrounding liquids [14]. In addition, the diameters of optical microfibers are much smaller than those of single-mode fiber, so microfibers have been adopted for high-precision sensing of external force [15]. All these features provide MLPGs may have application in highly sensitive optical sensing for strain, temperature, and refractive index [13,14,16–18].
Polymers exhibit many applications in optical sensing because of their sensitivity to ultraviolet [19], alcohol [20], humidity [21], and temperature [16], and to their biocompatibility [22]. Polymers on optical microfibers can be used for the fabrication of functional microstructures, without any damage of silica microfibers, which bestows them with much higher mechanical strength and flexibility. In particular, polymer jackets on microfibers under point-by-point ultraviolet exposure could enable an agile and versatile technique for flexible microstructure fabrication on optical microfibers.
Sign up for Photonics Research TOC. Get the latest issue of Photonics Research delivered right to you!Sign up now
In this work we demonstrate LPG inscription on optical microfibers functionalized with ultraviolet-sensitive polymers. We inscribe 2 mm long MLPGs with a pitch of 80 μm on a 5.4 μm diameter polymethyl methacrylate (PMMA)-coated microfiber via point-by-point ultraviolet exposure. A resonant dip of 15 dB was observed at 1377 nm. We also demonstrate possible applications in optical sensing with MLPGs. The strain sensitivity of the MLPG was measured to be and the resultant axial force sensitivity was calculated to be . Sealed by a PMMA housing, the temperature sensitivity of the MLPG could be increased to . The technique demonstrated here provides an agile and versatile technique for fabrication, and this highly sensitive MLPG could be used in optical sensing of strain, axial force, and temperature.
2. FABRICATION AND CHARACTERIZATION
Microfiber LPGs couple the fundamental mode and high-order modes in optical microfibers. This resonant coupling gives the phase-matching wavelength [23]:
The symbols and stand for the resonant wavelength and the grating pitch, respectively, and and for the effective refractive indices of the fundamental mode and high-order modes, respectively. In this work, we consider the grating pitch for the resonant coupling of the modes and . It can be calculated that the grating pitch is larger for a thicker microfiber.
In terms of strain sensing, the sensitivity of an MLPG is given by [23] where . Shown as the second term in Eq. (2), the strain sensitivity depends on the grating pitch. Although the greater grating pitch in a thicker microfiber would lead to a higher strain sensing sensitivity according to Eq. (2), the sensitivity of the axial force in a thicker microfiber could be reduces according to [15] where , , and are the applied axial force, the radius of microfibers, and the applied strain, respectively. The symbol is the Young’s modulus of silica, i.e., 73.1 GPa. To make a compromise between these considerations, we fabricated optical microfibers with a diameter of around 5 μm.
The optical microfibers were directly drawn from commercial single-mode fibers on a homemade taper drawing work station via the traveling flame taper-drawing scheme [24]. The diameters of the optical microfibers were measured to be around 5.4 μm and the insertion loss was less than 0.1 dB. To fabricate MLPGs, the optical microfibers were first coated with PMMA jackets via a modified dip-coating method [25]. The thickness of the PMMA jackets was estimated to be 100 nm under SEM photographs (not shown here), and the propagation loss was measured to be less than 0.5 dB/cm. We chose PMMA as the coating material because it can show remarkable refraction index changes and even photoetching under ultraviolet exposure [26,27].
The fabrication and measurement setup for MLPGs is shown in Fig. 1. We used a frequency-doubled argon laser at 244 nm for the inscription of MLPGs. The laser beam was focused by a fused silica lens and the focused spot diameter was about 30 μm. A translation stage was used to move the laser spot along the optical microfibers, and a shutter was adopted to control the exposure duration. The PMMA jacket was photoetched periodically by the ultraviolet laser, forming LPGs on optical microfibers. To characterize the MLPGs in real time during the inscription, we used a supercontinuum source (SCS) (SuperK COMPACT K90, NKT) for illumination, and the transmission spectrum was monitored on an optical spectrum analyzer (OSA) (86142B, Agilent).
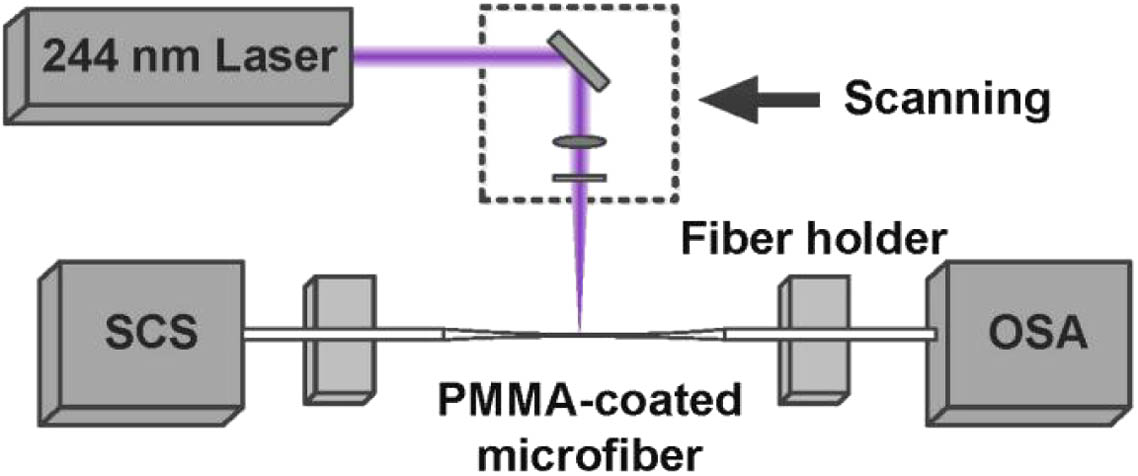
Figure 1.Schematic for the inscription of MLPGs via point-by-point ultraviolet exposure. A supercontinuum source (SCS) is used for illumination and the transmission spectrum is displayed on an optical spectrum analyzer (OSA) in real time.
In optical microfibers, the grating pitches of MLPGs (e.g., less than 100 μm) can be much shorter than the traditional LPGs [23] because of the relatively large differences between the propagation constants of the fundamental mode and high-order modes. The measured transmission spectrum of a 25-period MLPG is shown in Fig. 2(a). We obtained the optical spectrum of Fig. 2(a) by subtracting the transmission spectrum of coated optical microfiber after and before the inscription, to shown the clear spectrum induced by an MLPG that we intentionally inscribed. The grating pitch of the MLPG was 80 μm. and the total length of the MLPG was only 2 mm, which was about 1 order shorter than the traditional LPGs. The MLPG showed a 15 dB resonant dip at the wavelength of 1377 nm. Shown in Fig. 2(b) are the calculated resonant wavelengths of mode couplings for different grating pitches in the optical microfiber according to Eq. (1). For a grating pitch of 80 μm, the calculated resonant wavelength () between and matched well with the measured dip wavelength () of the 25-period MLPG.
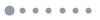
Figure 2.(a) Transmission spectrum of a 25-period MLPG. The grating pitch is 80 μm and the diameter of the optical microfiber is 5.4 μm. (b) The calculated grating pitches for different resonant dip wavelengths in optical microfiber with a diameter of 5.4 μm.
3. APPLICATIONS IN OPTICAL SENSING
The resonant wavelengths of MLPGs are sensitive to strain, temperature, and the refractive index of surrounding liquids. We measured the spectral responses to strain and temperature changes for the MLPGs and showed potential applications of MLPGs in optical sensing.
The measured spectral responses of the MLPG to strain are shown in Fig. 3(a). The strain was applied by stretching microfibers with a high-precision translation stage, ranging from 0 to 7533 μϵ. The measured resonant wavelengths under different strain are shown by the squares in Fig. 3(b), and the solid line is the linear fitting result. Evident blueshifts of the resonant wavelength were observed with the strain increase, and strain sensitivity of the MLPG was calculated to be . Note that this is 4 times larger than the LPGs in single-mode fibers [28]. The negative strain sensitivity may come from the photoelastic effect and the resultant negative value of [23].
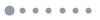
Figure 3.(a) Spectral responses of the MLPG to strain. The applied strain ranged from 0 to 7533 μϵ. (b) The measured resonant dip wavelengths (square scatters) and the linear fitting result (red line). The corresponding applied axial force is shown as the top axis.
In optical microfibers, the diameters are so small that a much higher sensitivity of axial force can be expected in MLPGs than that of LPGs in single-mode fibers, as shown in Eq. (3). Note that the impact of the PMMA jackets is negligible here, since the thickness of PMMA jackets is much smaller than the diameter of optical microfibers. The corresponding axial force is shown as the top axis in Fig. 3(b), and axial force sensitivity for the MLPG was calculated to be , according to Eq. (3), highlighted in Fig. 3(b). Compared with the single-mode fibers, the diameter of the MLPG is about 25 times smaller, so that the axial force sensitivity is more than 600 times higher than that of the LPGs in single-mode fibers. This high sensitivity to axial force of MLPGs promises potential applications in high-precision force sensing.
The spectral responses of the MLPG to temperature changes were measured with a digitally controlled oven. The temperature increased from 20°C to 50°C, and the transmission spectra of the MLPG at different temperatures are shown in Fig. 4(a). The resonant wavelengths showed slight blueshifts with increase of temperature, and the sensitivity was fitted to be about , as shown in Fig. 4(c). The relatively low temperature sensitivity of MLPGs can be explained by the small temperature sensitivity of pure silica in optical microfibers [13]. This low temperature sensitivity of MLPGs would be beneficial to reduce temperature-induced errors in the optical sensing of strain and axial force [29].
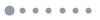
Figure 4.Spectral responses of (a) unsealed and (b) sealed MLPGs to temperature change. (c) The measured resonant wavelengths of sealed (square scatters) and unsealed (circle scatters) MLPGs at different temperatures. The linear fitting results are shown as red lines.
However, high temperature sensitivity of MLPGs is desirable for temperature sensing. To this end, we sealed the MLPG inside a PMMA housing that had a high thermal expansion coefficient. Single-mode fibers at the ends of optical microfibers were attached to the PMMA box, and the waist region was suspended in the air and kept straight in the box. The MLPG at the waist followed the expansion and shrinking of the PMMA housing caused by the temperature. In Fig. 4(b), we show the spectral responses of the sealed MLPG to temperature. It can be seen that the blueshifts of the resonant wavelength were increased significantly, and temperature sensitivity of the sealed MLPG was calculated to be , which is 30 times larger than that of the unsealed MLPG. It should be noted that the temperature sensitivity of the sealed MLPG is about 4 times larger than that of LPGs in the standard telecom fibers, about [28,30].
4. CONCLUSION
In conclusion, we have successfully inscribed LPGs on polymer functionalized optical microfibers via point-to-point ultraviolet exposure, and possible applications in optical sensing with the MLPGs are also demonstrated. For a 2 mm long MLPG with a pitch of 80 μm, the strain and axial force sensing sensitivity were and , respectively. Meanwhile, a relatively low temperature sensitivity of was observed for the unsealed MLPGs, which would be beneficial to reduce temperature-induced errors in optical strain and force sensing. For applications in temperature sensing, the sensitivity of the MLPG could be significantly enhanced to be by sealing inside a PMMA housing. Other than these demonstrations, MLPGs can also be combined with functional materials, such as polymers, graphene, and liquid crystal [12–14], promising applications in chemical and biological sensing.
Acknowledgment
Acknowledgment. This work is supported by National Natural Science Foundation of China (Grant No. 61505096).
References
[1] G. Brambilla, F. Xu, P. Horak, Y. Jung, F. Koizumi, N. P. Sessions, E. Koukharenko, X. Feng, G. S. Murugan, J. S. Wilkinson. Optical fiber nanowires and microwires: fabrication and applications. Adv. Opt. Photon., 1, 107-161(2009).
[2] L. Tong, F. Zi, X. Guo, J. Lou. Optical microfibers and nanofibers: a tutorial. Opt. Commun., 285, 4641-4647(2012).
[3] L. Tong, R. R. Gattass, J. B. Ashcom, S. He, J. Lou, M. Shen, I. Maxwell, E. Mazur. Subwavelength-diameter silica wires for low-loss optical wave guiding. Nature, 426, 816-819(2003).
[4] D. K. Armani, T. J. Kippenberg, S. M. Spillane, K. J. Vahala. Ultra-high-Q toroid microcavity on a chip. Nature, 421, 925-928(2003).
[5] X. Jiang, Y. Chen, G. Vienne, L. Tong. All-fiber add-drop filters based on microfiber knot resonators. Opt. Lett., 32, 1710-1712(2007).
[6] Y. Li, L. Tong. Mach-Zehnder interferometers assembled with optical microfibers or nanofibers. Opt. Lett., 33, 303-305(2008).
[7] X. Guo, Y. Ying, L. Tong. Photonic nanowires: from subwavelength waveguides to optical sensors. Acc. Chem. Res., 47, 656-666(2013).
[8] J. Kou, M. Ding, J. Feng, Y. Lu, F. Xu, G. Brambilla. Microfiber-based Bragg gratings for sensing applications: a review. Sensors, 12, 8861-8876(2012).
[9] G. Y. Chen, M. Ding, T. P. Newson, G. Brambilla. A review of microfiber and nanofiber based optical sensors. Open Opt. J., 7, 32-57(2013).
[10] F. Pang, X. Sun, H. Guo, J. Yan, J. Wang, X. Zeng, Z. Chen, T. Wang. A PbS quantum dots fiber amplifier excited by evanescent wave. Opt. Express, 18, 14024-14030(2010).
[11] Z. Xu, Y. Li, L. Wang. Versatile technique to functionalize optical microfibers via a modified sol-gel dip-coating method. Opt. Lett., 39, 34-36(2014).
[12] W. Li, B. Chen, C. Meng, W. Fang, Y. Xiao, X. Li, Z. Hu, Y. Xu, L. Tong, H. Wang, W. Liu, J. Bao, Y. R. Shen. Ultrafast all-optical graphene modulator. Nano Lett., 14, 955-959(2014).
[13] H. Xuan, W. Jin, S. Liu. Long-period gratings in wavelength-scale microfibers. Opt. Lett., 35, 85-87(2010).
[14] H. Xuan, W. Jin, M. Zhang. CO2 laser induced long period gratings in optical microfibers. Opt. Express, 17, 21882-21890(2009).
[15] W. Li, Z. Hu, X. Li, W. Fang, X. Guo, L. Tong, J. Lou. High-sensitivity microfiber strain and force sensors. Opt. Commun., 314, 28-30(2014).
[16] X. Zhang, Y. Yu, C. Chen, C. Zhu, R. Yang, Z. Liu, J. Liang, Q. Chen, H. Sun. Point-by-point dip coated long-period gratings in microfibers. IEEE Photon. Technol. Lett., 26, 2503-2506(2014).
[17] C. L. Lee, Z. Y. Weng, C. J. Lin, Y. Lin. Leakage coupling of ultrasensitive periodical silica thin-film long-period grating coated on tapered fiber. Opt. Lett., 35, 4172-4174(2010).
[18] G. Kakarantzas, T. Birks, P. St. J. Russell. Low-loss deposition of solgel-derived silica films on tapered fibers. Opt. Lett., 29, 694-696(2004).
[19] Z. Chen, V. K. S. Hsiao, X. Li, Z. Li, J. Yu, J. Zhang. Optically tunable microfiber-knot resonator. Opt. Express, 19, 14217-14222(2011).
[20] Y. S. Chiam, K. S. Lim, S. W. Harun, S. N. Gan, S. W. Phang. Conducting polymer coated optical microfiber sensor for alcohol detection. Sens. Actuators A, 205, 58-62(2014).
[21] L. Zhang, F. Gu, J. Lou, X. Yin, L. Tong. Fast detection of humidity with a subwavelength-diameter fiber taper coated with gelatin film. Opt. Express, 16, 13349-13353(2008).
[22] L. Bo, C. C. O’Mahony, Y. Semenova, N. Gilmartin, P. Wang, G. Farrell. Microfiber coupler based label-free immunosensor. Opt. Express, 22, 8150-8155(2014).
[23] S. W. James, R. P. Tatam. Optical fibre long-period grating sensors: characteristics and application. Meas. Sci. Technol., 14, R49-R61(2003).
[24] Y. Li, Y. Zhao, L. Wang. Demonstration of almost octave-spanning cascaded four-wave mixing in optical microfibers. Opt. Lett., 37, 3441-3443(2012).
[25] Z. Xu, Y. Li, L. Wang. In situ fine tailoring of group velocity dispersion in optical microfibers via nanocoatings. Opt. Express, 22, 28338-28345(2014).
[26] Y. Kawamura, K. Toyoda, S. Namba. Effective deep ultraviolet photoetching of polymethyl methacrylate by an excimer laser. Appl. Phys. Lett., 40, 374-375(1982).
[27] Y. Ichihashi, P. Henzi, M. Bruendel, J. Mohr, D. G. Rabus. Polymer waveguides from alicyclic methacrylate copolymer fabricated by deep-UV exposure. Opt. Lett., 32, 379-381(2007).
[28] Y. J. Rao, Y. P. Wang, Z. L. Ran, T. Zhu. Novel fiber-optic sensors based on long-period fiber gratings written by high-frequency CO2 laser pulses. J. Lightwave Technol., 21, 1320-1327(2003).
[29] Y. P. Wang, L. Xiao, D. N. Wang, W. Jin. Highly sensitive long-period fiber-grating strain sensor with low temperature sensitivity. Opt. Lett., 31, 3414-3416(2006).
[30] V. Bhatia. Applications of long-period gratings to single and multi-parameter sensing. Opt. Express, 4, 457-466(1999).