Abstract
We report the modulation of epsilon-near-zero (ENZ) wavelength and enhanced third-order nonlinearity in indium tin oxide (ITO)/Au multilayer films. The samples consisting of five-layer 40 nm ITO films spaced by four-layer ultrathin Au films of different thickness, i.e., ITO(40 nm)/[Au(x)/ITO(40 nm)]4, were prepared by magnetron sputtering at room temperature. The ENZ wavelength in the multilayer films is theoretically calculated and experimentally confirmed. The nonlinear refractive index and nonlinear absorption coefficient of the samples of x, 2, 3, 4 nm were determined using the Z-scan method at a wavelength of 1.064 µm. The large nonlinear refractive index n2 and nonlinear absorption coefficient in the sample of x = 4 nm are both four times larger than those in the single-layer ITO film. The large optical nonlinearity due to the ENZ enhancement and carrier concentration is discussed. The results indicate that the ITO/Au multilayer films are promising for advanced all-optical devices.1. Introduction
Material that possesses strong and ultrafast nonlinear optical response and is compatible with the complementary metal-oxide semiconductor (CMOS) fabrication technologies is essential for the high-performance integrated on-chip nonlinear optical devices, such as all-optical signal processing, computing, and communicating[1]. Recently, materials of free charges were widely reported to possess the extremely large third-order nonlinearity at their epsilon-near-zero (ENZ) frequencies, which show their promise for applications in nonlinear optics[2]. Indium tin oxide (ITO), as one of the typical CMOS-compatible and ENZ materials working at near-infrared, draws more attention since the extremely large effective nonlinear refractive index and nonlinear absorption coefficient measured at angle of incidence 60° were found to be around 43 and 45 times larger than those obtained at the normal incidence[3]. The nonlinear refractive index as large as was further obtained at the ENZ wavelength in the Au nanoantennas coupled to ITO films[4]. The large nonlinear refractive index () and nonlinear saturable absorption coefficient () were also recently obtained in ITO/Ag/ITO sandwiches[5].
However, the ENZ wavelength of ITO and other ENZ materials, is governed by the intrinsic material properties and occurs at a special regime[6], which limits their applications for photonic devices working at a broad band. Though the ENZ wavelength of ITO can be modulated by controlling the fabrication parameters[7], it is still working limitedly in the near-infrared wavelength regime, which is determined by the intrinsic properties. The layered metal-dielectric multilayer stacks offer an effective and simple way to tune the effective ENZ wavelength[8]. The effective permittivity of multilayer stacks can be calculated using the formula when the polarization of the electric field is parallel to the plane of the layers, where refer to the volume fraction of material and material with ; and are the permittivities of layers and , respectively[9]. The combination of the noble metals possessing ENZ behavior in the UV region and particular dielectrics of ENZ behavior in the infrared region can extensively modulate the effective ENZ wavelength covering from the UV to infrared region in such composites and further modify the effective optical nonlinearity in a wide band for nonlinear devices. Though the enhanced third-order optical nonlinearity in layered metal-dielectric multilayer stacks with ENZ behavior at visible wavelength has been reported by Suresh et al.[8], the dielectric materials of negligible nonlinear response were used, and only the nonlinearity from Ag is involved. The dominant optical nonlinearity in the dielectric layer in multilayer films of tunable ENZ wavelength is still lacking investigation.
In this Letter, we report the modulation of ENZ wavelengths and third-order nonlinearity in ITO/Au multilayer films. The ENZ wavelength was tuned to be around 0.94 µm and 1.29 µm in the multilayers consisting of five-layer ITO film (each layer thickness 40 nm) and inserted four-layer Au of each layer thickness of 3 and 4 nm, respectively. The large nonlinear refractive index and nonlinear absorption coefficient measured at 1.064 µm were obtained, which are four times larger than those in the pure ITO film. The results show that the ITO/Ag multilayer films are promising candidate materials for all-optical devices working at a desired wavelength.
Sign up for Chinese Optics Letters TOC. Get the latest issue of Chinese Optics Letters delivered right to you!Sign up now
2. Experimental Details
The theoretical effective permittivity of multilayer stacks based on the reported dielectric constant of ITO[3] and Au[10] is shown in Fig. 1(a). It is clear that the ENZ wavelength of ITO/Au multilayer films can obviously be modulated with the increase of the filling factor of Au . Experimentally, the ITO/Au/ITO (IAI) multilayer films and pure ITO films were prepared on fused silica substrates () at room temperature using the magnetron sputtering method. The base pressure of the chamber was pumped to be , and the substrate was rotated at a uniform speed of 15 revolutions per minute to ensure uniform film thickness during the deposition. During the deposition, the DC power was kept at 120 W and Ar flow as 20 sccm (standard cubic centimeters per minute). The deposition rate is around 1 nm/s. The ITO target was a 10% (mass fraction) -doped disk (purity 99.99%), and an Au target of purity 99.99% was used for the deposition. The multilayer films consist of five-layer 40 nm ITO films and four-layer Au films of different thickness of , 3, and 4 nm inserted between the ITO layers, i.e., , denoted as IAI-2, IAI-3, and IAI-4, respectively. The schematic structure of the multilayer film is shown in Fig. 1(b). The pure ITO film of thickness 200 nm was fabricated and denoted as pure ITO. The growth rate of each layer was determined by the quartz crystal oscillator, and the desired thickness of each was controlled by the sputtering time, which was further examined by scanning electron microscopy (SEM).
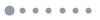
Figure 1.(a) Theoretical real part of effective permittivity of ITO/Au multilayer stacks. (b) Schematic diagram of an ITO-Au multilayer stack. (c) Cross-sectional image and (d) surface morphology of the IAI-4 multilayer film obtained by SEM.
3. Results and Discussion
The cross section of the IAI-4 multilayer film measured by SEM is shown in Fig. 1(c). The interfaces between ITO and Au are observed, and the thickness of each layer is uniform. The total thickness is measured to be around 221 nm, which is close to the expected thickness of 216 nm controlled by the growth rate and sputtering duration. The surface morphology of the IAI-4 film in Fig. 1(d) indicates that the film has a very compact and uniform surface in the large scale. No cracks and large particles were observed on the surface of the film.
The crystallization of the pure ITO and IAI multilayer films was characterized by X-ray diffraction (XRD). The XRD patterns of the pure ITO and IAI-4 films are shown in Fig. 2. The diffraction peaks in the pure ITO film indicate the 200 nm ITO film has a polycrystalline structure. However, there are no obvious peaks from ITO in the IAI-4 multilayer film. This is probably ascribed to the fact that the crystallization of ITO is influenced by the thickness. During the growth, the accumulation of sputtering may help to materialize the crystallization due to the tension, and thus the polycrystalline structure is formed in 200 nm ITO film, but not in 40 nm ITO film. The Au (222) peak is clearly observed in the IAI-4 film. The crystallite size of Au is estimated to be about 5.2 nm, which is close to the nominal thickness of 4 nm, using the Scherrer equation , where is the average crystallite size in the direction perpendicular to the reflecting plane, is a constant at 0.89, is the X-ray wavelength, is the full width at half maximum of the diffraction peak, and is the diffraction angle.
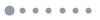
Figure 2.XRD patterns of the pure ITO film and IAI-4 multilayer film.
The refractive index and extinction coefficient of the films were determined by an ellipsometer in the range of 0.6–1.7 µm. The permittivity is obtained from the refractive index and extinction coefficient , which were determined from ellipsometer data by . The real () and imaginary () parts of dielectric permittivity in pure ITO film and the effective permittivity of the multilayer films are shown in Fig. 3. Note that the ENZ wavelength of the pure ITO film would beyond 1.7 µm, which is out of the range of our ellipsometry instrument. It is probably due to the atom derivation and microstructures in the films drawn by the preparation parameters, which was also found in Ref. [7]. When the Au layers are inserted in the ITO/Au multilayer stacks, the permittivity is effectively modulated. When Au layer is 2 nm, the ENZ wavelength is still beyond 1.7 µm. The ENZ wavelength is clearly observed and is around 0.94 µm and 1.29 µm when the Au layer achieves 3 nm and 4 nm, respectively. The results show that the ENZ wavelength is indeed modulated in the multilayer stacks by changing the fill factor of noble metal. The change agrees well with the theoretical prediction [Fig. 1(a)], i.e., the increase of Au volume fraction will lead to the blueshift of ENZ wavelength. The ENZ wavelength could be further blue-shifted with the increase of the thickness of the Au layer and fall into the visible region. Note that the imaginary part of effective permittivity of multilayer films significantly increased compared to the pure ITO film, which is detrimental to the photonic devices. Next, we will see that the large does not affect the enhancement of optical nonlinearity, but is still a worthy concerned parameter for the design of photonic devices.
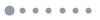
Figure 3.Experimentally determined permittivity of the pure ITO film and effective permittivity of the multilayer films.
The third-order optical nonlinearity of the samples was investigated using a single-beam Z-scan method[11]. The typical Z-scan experimental setup for measurements is similar to that used in our previous works[12,13]. In brief, a (Nd:YAG) laser with a wavelength of 1.064 µm and pulse duration of 25 ps was used as the light source. The focal length of the lens before the samples was 150 mm, which leads to the radius of the beam waist around 40 µm and the peak intensity around . The power of the reference beam and the transmitted beam was recorded by the detectors connected to the power meter (PM320E, Thorlabs Inc.), respectively. The linear transmission of the far field was set to be 0.2. For each sample, the Z-san measurement was conducted at 20 different positions to ensure the homogeneity of the samples. The uncertainty of the determined values of nonlinear absorption and refractive index is around 20%. The representative normalized transmittances, as well as their theoretical fitting curves, of the samples measured under the open-aperture (OA) and closed-aperture (CA) Z-scan method are shown in Fig. 4. The nonlinear optical response of the fused silica substrate is quite weak and negligible under such laser intensity, so the patterns of OA and CA measurements are from the samples. The peak patterns of OA Z-scan curves in the samples indicate that all samples possess saturation absorption. The CA Z-scan curves of the samples all show valley-peak patterns, indicating that the samples have self-focusing effects and positive nonlinear refractive index. The calculated values of and are given in Table 1. It is noted that the values in this work are much larger than those reported in the references, which is ascribed to the probably involved thermal nonlinearity due to the employed picosecond-pulsed laser source.
Sample | β (×10−8 m/W) | n2 (×10−14 m2/W) | Carrier Concentrations (×1021 cm−3) |
---|
Pure ITO | −4.99 | 2.55 | 0.13 |
IAI-2 | −11.64 | 8.01 | 1.13 |
IAI-3 | −13.89 | 11.16 | 4.98 |
IAI-4 | −17.80 | 11.21 | 6.86 |
Table 1. Saturable Absorption Coefficient β, Nonlinear Refractive Index n2, and Carrier Concentrations in the Samples
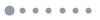
Figure 4.(a) OA and (b) CA Z-scan data with the theoretical fittings for pure ITO film and multilayer films.
It is obvious that the nonlinear optical response in the multilayer films is efficiently enhanced compared with that in the pure ITO film. The saturable absorption coefficient and nonlinear refractive index achieve their maximum values in the IAI-4 film. Compared to the and in the pure ITO film, the and in the IAI-4 film both increase around four times. The optical nonlinearity of Au film at 1.064 µm is too weak to exhibit the typical OA and CA Z-scan patterns below the laser damage threshold. Given that the nonlinearity of the multilayer films satisfies the nonlinear effective medium theory, i.e., , where , , and are the effective third-order nonlinear susceptibilities of the composite material and materials and , respectively. If the nonlinearity of Au is neglected, the multilayer films have the effective third-order nonlinear susceptibility . Since , the ITO/Au multilayer films will give a smaller than that of the pure ITO film. However, the values of in multilayer films are larger than that in the pure ITO film, especially, the of IAI-3 and IAI-4 films, which both possess the ENZ wavelength closer to 1.064 µm that is four times larger than that of pure ITO film, along with the . Such enhancement is mainly ascribed to the ENZ effect. The refractive index change follows the relation [3], where and are the change of dielectric permittivity and permittivity of materials, respectively. The achieves its maximum value at the zero-permittivity wavelength. In the multilayer films, the complex nonlinear response of the composite films is written as , where is the vacuum permittivity, is the speed of light in the vacuum, and is the refractive index of composite film. The reduced near the ENZ wavelength will increase the , as reported in Ref. [8].
To further explore the causes of the optical nonlinearity enhancement, the carrier concentrations and resistivity measured by the four-probe Hall method are given, as shown in Table 1 and Fig. 5. The pure ITO film has the highest resistivity () and the lowest carrier concentration (). The values are effectively modified in the multilayer films with the introduction of Au layers. As the Au layer increases from 2 to 4 nm, the resistivity monotonically drops to , and the carrier concentration rises to . Note that the values of resistivity and carrier concentration are quite close to those reported in Ref. [5]. The and synchronously change with carrier concentration, which indicate that besides the modulation of the ENZ wavelength the ITO/Au multilayer films also tune the carrier concentration to influence the optical nonlinearity and agree with the conclusions in Refs. [5,14]. The change of concentration in the multilayer films with the different thickness of the Au layer may be ascribed to the electron transport property and interface effect between the ITO and Au layers, as discussed in Ref. [5]. With the increase of the Au layer, the carrier mobility of the composite films will increase due to the low resistivity of thick bulk metal.
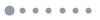
Figure 5.Carrier concentration, resistivity, and nonlinear optical response versus the thickness of the Au layer.
We noticed that there exists the large enhancement of the local field between the Ag islands to enhance the optical nonlinearity in ITO/Ag/ITO films[5]. We conducted the numerical simulation to obtain the local field distribution for our samples. However, no enhancement was found, even in the sample of the 4 nm Au layer. The reason may be that the Au particle is too small at the nonresonant wavelength of 1.064 µm.
4. Conclusions
In summary, we modulated the ENZ wavelength in the ITO/Au multilayer films by changing the thickness of the Au layer and confirmed the enhanced third-order nonlinearity in ITO/Au multilayer film at around the ENZ wavelength. The combination of noble metal and dielectric film possessing the ENZ wavelength at the infrared wavelength theoretically can tune the effective ENZ wavelength covering the UV to infrared regions, though the imaginary part of effective permittivity in multilayers should be balanced for optimized designs. The enhanced optical nonlinearity at the designed ENZ wavelength indicates that such multilayer stacks offer a flexible platform for advanced all-optical devices.
References
[1] R. W. Boyd. Nonlinear Optics(2003).
[2] O. Reshef, I. De Leon, M. Z. Alam, R. W. Boyd. Nonlinear optical effects in epsilon-near-zero media. Nat. Rev. Mater., 4, 535(2019).
[3] M. Z. Alam, I. De Leon, R. W. Boyd. Large optical nonlinearity of indium tin oxide in its epsilon-near-zero region. Science, 352, 795(2016).
[4] M. Z. Alam, S. A. Schulz, J. Upham, I. De Leon, R. W. Boyd. Large optical nonlinearity of nanoantennas coupled to an epsilon-near-zero material. Nat. Photonics, 12, 79(2018).
[5] K. Wu, Z. W. Wang, J. H. Yang, H. Ye. Large optical nonlinearity of ITO/Ag/ITO sandwiches based on Z-scan measurement. Opt. Lett., 44, 2490(2019).
[6] I. Liberal, N. Engheta. Near-zero refractive index photonics. Nat. Photonics, 11, 149(2017).
[7] A. Capretti, Y. Wang, N. Engheta, L. Dal Negro. Comparative study of second-harmonic generation from epsilon-near-zero indium tin oxide and titanium nitride nanolayers excited in the near-infrared spectral range. ACS Photonics, 2, 1584(2015).
[8] S. Suresh, O. Reshef, M. Z. Alam, J. Upham, M. Karimi, R. W. Boyd. Enhanced nonlinear optical responses of layered epsilon-near-zero metamaterials at visible frequencies. ACS Photonics, 8, 125(2021).
[9] R. W. Boyd, J. E. Sipe. Nonlinear optical susceptibility of layered composite materials. J. Opt. Soc. Am. B, 11, 297(1994).
[10] P. B. Johnson, R. W. Christy. Optical constants of the noble metals. Phys. Rev. B, 6, 4370(1972).
[11] M. Sheik-Bahae, A. A. Said, T. H. Wei, D. J. Hagan, E. W. Van Stryland. Sensitive measurement of optical nonlinearities using a single beam. IEEE J. Quantum Electron., 26, 760(1990).
[12] T. Ning, H. Lu, Y. Zhou, B. Man. Decrease and enhancement of third-order optical nonlinearity in metal-dielectric composite films. Appl. Phys. Lett., 112, 151904(2018).
[13] T. Ning, Y. Zhou. Effect of structure on nonlinear optical properties in CaCu3Ti4O12 films. J. Appl. Phys., 118, 233103(2015).
[14] H. I. Elim, W. Ji, F. Zhu. Carrier concentration dependence of optical Kerr nonlinearity in indium tin oxide films. Appl. Phys. B, 82, 439(2006).