
- Chinese Optics Letters
- Vol. 13, Issue Suppl., S21501 (2015)
Abstract
Laser range finders that employ time-of-flight techniques can be divided into three categories: pulsed techniques[
The principle of the phase difference method is shown in Fig.
Figure 1.Block diagram of the phase difference laser range finder.
The resolution of the distance is given by
Sign up for Chinese Optics Letters TOC. Get the latest issue of Chinese Optics Letters delivered right to you!Sign up now
It is difficult to improve the resolution of the phase difference
First, we analyze the influence of the electrical crosstalk on the phase difference in the following. The error expression given illustrates that the crosstalk will yield a periodic phase difference errors versus the distance
When the photoelectric signal (
Then, the actual measured signal is given by
Using Eqs. (
Therefore
The influences of the crosstalk on the distance as derived from Eq. (
Figure 2.The influences of the crosstalk on the phase difference and distance, for
Figure 3.The actual periodic errors of the range system.
It seems that there are some differences between Eqs. (
In Eq. (
Using an APD as an optoelectronic mixer can eliminate the periodic error[
The phase drift may lead to irregular phase difference errors, which are still rather difficult to implement. These are mainly caused by three factors: frequency drift[
The condition of conjugate impedance matching for every basic power transportation unit of the circuits not only enables maximum power transportation but also eliminates phase shift when the power is transported from the source to the load. This is due to the fact that the resulting impedance contains the only pure resistance in the entire source-load circuit loop under the condition of conjugate impedance matching. In an unmatched case, the phase shift in the circuits is a function of the impedance and frequency. If a perfect symmetry is obtained between the reference signal and the work signal circuits, it can be supposed that the main oscillator frequency drift or the local oscillator frequency drift balances the drift of the other one. In this case, no error is present, as the reference signal channel and the work signal channel are identical. However, it is difficult to fulfill exact matching and symmetry in actual circuit boards. Thus, the frequency or the impedance drift produce a phase difference drift so as to impact the performance of the range finder. A phase difference drift obtained with the range system described in Fig.
Figure 4.Phase difference drift.
In actual oscillators, the circuit and system noise gives rise to phase noise and random jitter. The phase difference drift caused by the noise can be reduced by rejecting the common mode noise using a differential pair runner, eliminating spuriousness by filtering, and calculating the phase difference using the multi-cycle cross-correlation method[
The block diagram of our proposed phase difference range finder is shown in Fig.
Figure 5.Block diagram of the proposed phase difference laser range finder.
The modulation unit produces the sinusoidal modulation signal. The main oscillator synthesizes 1.4985 and 1.4835 GHz signals sequentially with digital fractional phase-locked loops (we used the ADF4350 wideband synthesizer) from an analog device. The first frequency led to a 2.8 μm distance resolution with a phase measurement resolution of 0.01°, while the unambiguous range with respect to the difference of the two frequencies is 10 m. The intensity of the laser beam of the 1550 nm laser with a power of 40 mW is modulated by a Mach–Zehnder modulator. Experiments show that if the main oscillator is independent to the local oscillator, a large drift in the intermediate frequency may occur. The local oscillator in the processing unit has the same configuration as the main oscillator, but their frequencies are different. To reduce the frequency drift of the intermediate frequency signals, both oscillators are driven by the same temperature-compensated crystal.
The emission and detection parts that are associated with the optical components form the optics unit. The distance to be measured is indicated by the zero position and the target position. The round-trip time of the modulated beam between the zero position and the target position is proportional to the phase delay of the wave. But a large error occurs when the phase delay is measured directly due to the phase difference drift, which is caused by frequency drift and the impedance drift of the circuits. In the optics unit, a differential measurement method is used to eliminate the phase difference drift by introducing the optical switch. The optical switch and the oscillators are controlled by a single-chip microcomputer, which is not shown in Fig.
In photoelectric modulator 2, the light is modulated again by sine signals generated by the local oscillator. That means that modulator 2 worked as a mixer. The signal frequency of the local oscillator is always 1 MHz less than that of the main oscillator, so the frequency of the photoelectric signal output from the photodetector is different from the signal frequency of the main oscillator, and the crosstalk is eliminated.
The phase difference estimation and the distance calculation are realized by the processing unit. Precisely measuring the phase difference at about 1.5 GHz is quite difficult. The heterodyne technique that preserves the phase difference versus the distance allows the phase difference to be measured at an intermediate frequency. One intermediate frequency signal has been obtained from the photodetector. Another reference signal at 1 MHz is obtained by mixing the signals that come directly from the main oscillator and the local oscillator. Both intermediate frequency signals are sampled synchronously by a dual-channel 48 MHz analog-to-digital converter. Finally, the phase difference is estimated using a correlation analysis, and the distances are calculated by the computer.
The phase difference drift has been examined when the modulation frequency and the distance remain unchanged. The results are shown in Fig.
Figure 6.Phase difference drift of the modified system.
Figure
Figure 7.Experimental setup.
Figure 8.Distance error of the range finder.
In conclusion, based on the phase difference range finder, a high-frequency range system for improving the accuracy of the phase difference is presented. The analyses and experiments demonstrate that the periodic errors with a period of a half wavelength of the modulation signal caused by the electrical crosstalk and the phase difference drift produced by the frequency and impedance drift will give rise to notable phase difference errors. In our system for reducing the phase difference drift, both the main oscillator and the local oscillator are synthesized by digital fractional phase-locked loops, and a differential measurement method is used by introducing the optical switch. To eliminate the electrical crosstalk between the photoelectric and the modulation signals, a photoelectric modulator is used as a mixer. Compared with the distance value of the laser interferometer, the error of the proposed range system is within
References
[1] S. Kruapech, J. Widjaja. Opt. Laser Technol., 42, 749(2010).
[2] F. Yang, Y. He, J. H. Shang, W. B. Chen. Appl. Opt., 48, 6575(2009).
[3] I. Fujima, S. Iwasaki, K. Seta. Meas. Sci. Technol., 9, 1049(1998).
[4] S. Liu, J. Tan, B. Hou. Meas. Sci. Technol., 18, 1756(2007).
[5] D. Dupuy, M. Lescure, H. Tap-Béteille. Sens. Actuators A: Phys., 110, 289(2004).
[6] C. A. Cifuentes, A. Frizera, R. Carelli, T. Bastos. Rob. Auton. Syst., 62, 1425(2014).
[7] A. M. Pinto, L. F. Rocha, A. Paulo Moreira. Rob. Comput. Integr. Manuf., 29, 12(2013).
[8] D. Castagnet. Opt. Eng., 45, 043003(2006).
[9] T. Bosch. Opt. Eng., 40, 10(2001).
[10] Y. R. Liang, H. Z. Duan, H. C. Yeh, J. Luo. Rev. Sci. Instrum., 83, 095110(2012).
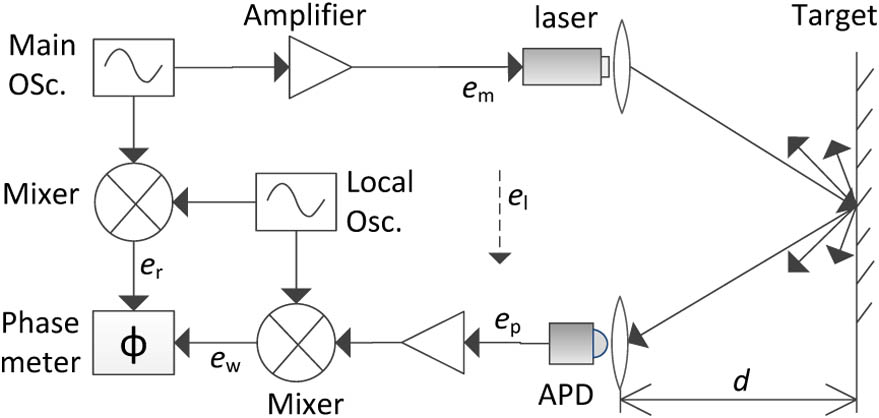
Set citation alerts for the article
Please enter your email address