
- Journal of Resources and Ecology
- Vol. 11, Issue 4, 342 (2020)
Abstract
Keywords
1 Introduction
In a broad sense, timberline, or forest limit, is the upper limit of forest on a high-elevation mountain (
Timberline is not an abruptly physical line, but rather a boundary or transition zone. Usually the upper limit of climatic closed forests with a steep gradient of increasing stand fragmentation and stuntedness, is called as treeline ecotone (
The Himalayas is a unique physic-geographical region with average elevation higher than 4000 m. Monsoon and westerlies alternatively influence the climate across the Himalayas (
Many studies have been carried out on the vertical vegetation zonation and spruce-fir forest over the past 30 years (
The main objectives of this review are to describe floristic patterns of treeline species and the spatial distribution of treeline in the Himalaya region, identify its relation to climatic conditions, and summarize the mechanisms of treeline formation and the response of tree growth and regeneration at treeline ecotone to climate change.
2 Tree species floristic at timberline in Himalayan region
2.1 Floristic patterns of timberline tree species
Spruce-fir forests are distributed mainly in the southeastern part of the Tibetan Plateau, extending between 85-105°E and 26-38°N. In the southeastern part of the Tibetan Plateau and southern slope of the Himalayan Range, the coniferous species are diverse. It is reported that there are 16 species of Abies, 16 species of Picea, six species of Larix and 11 species of Juniperus (Sabina) on the Plateau. But only 14 species of genus Abies, five of Picea, five of Juniperus and four of Larix can reach climatic forest limit and consist of timberline species. In addition, schlerphyllous Quercus and deciduous broad-leaved trees, e.g. Betula, etc. can also form forest limit vegetation in west Himalaya, southeast Tibet and north Hengduan Mountaints. For example, Betula utilis presents at treeline in Uttarakhand, India. The Hengduan Mts. serve as species differentiation center of Abies. There are nine species of Abies in this region, of which Abies ferreana, A. squamata, A. nukiangensis, A. delavayi, A. georgei, A. georgei var. smithii, and A. forrestii constitue forest limit species. Kangding, in western Sichuan Province, is the species differentiation center of genus Picea and there covers over 10 species of spruce forests of the Qinghai-Tibet Plateau. But only some species such as Picea balfouriana, P. purpurea, P. likiangensis and P. crassifolia can reach forest limit and form timberline, in which P. balfouriana is the most common and widely distributed timberline species in eastern Tibet and northwestern Sichuan plateau. Larix is the forest limit genus on sunny slopes. In western Sichuan, L. potaninii is the widespread timberline species and can extend to Bailongjiang watershed of southern Gansu Province. L. potanini var. marcrocarpa is widely distributed in southwestern Sichuan, northwestern Yunnan and northeastern Tibet. At the elevation of 3800-4300 m, forest limit forms together with Aibes. Sabina (now Juniperus) is the alpine tree species and is the highest forest type to constitute forest limit. S. convallium, S. sultuaria and S. tibetica are the timberline species on sunny slopes in western Sichuan and eastern Tibet. In eastern Qinghai, southern Gansu and northwestern Sichuan, S. przewalskii, and S. komarovii are dominant timberline species on sunny slopes (
Figure 1.Fig. 1
On southern slopes of the Himalayas, Juniperus indica, J. recurra and Abies spectabilis, A. densa, A. pindrow as well as Betula utilis occur at treelines (
Figure 2.Fig. 2
2.2 Distribution patterns of timberline altitude on the Qinghai-Tibet Plateau
(1) The elevation of timberline distribution on the Qinghai-Tibet Plateau
In the southeastern part of the Qinghai-Tibet Plateau, the elevation of mountains decreases gradually with the advance of longitude. In general, the elevation of timberline increases from 3600 m in the western bordering mountains of Sichuan Basin to 4300 m in eastern Tibet and northwestern Sichuan plateau. In east Tibet and north section of the Hengduan Mts., Picea balfouriana and Abies squamata constitute the highest timberlines of spruce-fir forests in the world. The timberlines can reach 4300 m and even higher in the areas. Towards southeast, in the Sichuan Basin bordering mountains from Tianquan, Baoxing to Jiuzhaigou, Abies faxoniana, A. faberi and Picea purpurea grow on 3500- 3800 m to form timberline. 30°N is the natural boundary of spruce-fir species differentiation and their distribution. It is apparent that the elevation of timberline gradually increases with the decrease of latitude on the Qinghai-Tibet Plateau. The elevations of timberlines reach the highest position in 30°N. Northward from this latitude, the elevation of timberline begins to decrease (
(2) The patterns of timberline distribution
The elevations of timberline distribution along latitude and longitude are influenced markedly by the uplifting of the Qinghai-Tibet Plateau and vary complicatedly and irregularly on the Plateau. Some scientists named it as “plateau vegetation zonation” (
The latitude of timberline increases with the uplifting of the Plateau from east to west. The altitude of timberline distribution along longitude is approximate to parabola from 86-104°E. The peak area of timberline distribution is in the scope of 92-97°E. It is situated in the areas around the turn of the Yarlung Zangbo River and its tributary Polong Zangbo River, in eastern Tibet. From southeastern Tibet to the west, i.e., the southern slope of the Himalayas, the altitudes of timberlines are basically stable at the latitude of 4000-4100 m. From eastern Tibet to western Sichuan plateau, the elevations of timberlines decrease gradually and decline approximately in a form of straight line to the western bordering mountains of the Sichuan Basin.
The elevations of timberline distribution across latitude ranging from 26°E to 38°E on the Qinghai-Tibet Plateau can be simulated by polynomial regression model. The peak areas of timberline distribution are around the latitude of 30°E.
Three dimensional distribution of timberline along longitude and latitude can be modeled by surface analysis. It is indicated that the distribution of timberline elevation on the Plateau is in inverse “S” shape along latitude and longitude. The surface model of timberline distribution is:
where H is the elevation of timberline, x is longitude and y is latitude. The timberline distribution reaches the highest elevation at 92.48°E and 31.32°N. It is right in the eastern part of Tibet that the Grand Canyon of the Yarlung Zangbo River is located.
3 Climatic factors influence timberline distribution
Temperature is a well-recognized predictor of timberline position and distribution in global scale. However, there are few weather stations established near timberline. A lot of temperature extrapolation from lower or nearby weather station was used as a proxy of climatic conditions at timberlines despite its bias. Therefore, there exist uncertainties because of topographical and vegetation status over there. The most acknowledged is that 10℃ warmest month isotherm was observed to represent the geographical location of the world timberline.
The air temperature of timberline shows great variation in different region.
Currently on-site observed ca. 6.5℃ of mean growing seasonal temperatures at depth of 10 cm soil, varies with very little site to site variation. The seasonal mean temperature has been proved as a better predictor of treeline position than warmest month temperatures or a suite of thermal sums tested (
Although soil temperature plays a profound role in seedling establishment, tree growth and survival, very few measurements of soil temperatures exist at treelines in the HKH mountains. More studies are necessary to be conducted in between treeline tree growth and soil temperature.
4 Water, nutrient and carbon supply in treeline ecotone
It is assumed that nutrient becomes limitation in high altitudinal treeline because nutrient availability is constrained by low temperature. But there are two facts against this assumption. First, the nutrient contents, especially nitrogen, in organisms at treelines are never less than those in closed forest in lower altitude (
The debate over the mechanistic factors that limit the altitudinal treeline has been continued for over a century (
5 Impact of global warming on treeline change
The Himalayas experienced increasing warming in the past two decades. Himalayas have warmed by 1.5℃ from 1982 to 2006, at an average rate of 0.06 ℃ yr-1. Temporal differentiation present seasonal difference with greatest average increase of 0.07 ℃ yr-1 in winter, and least increase, 0.03 ℃ yr-1 in summer (
Monitoring treeline shift response to climate change is possible with the use of “an eye in the sky” (i.e. remote sensing satellite) in the inaccessible Himalayan terrain (
However, in-site observation is few due to remoteness and costing. In east Himalayas, treeline shift is not apparent. Most of treeline vegetation change is growth and regeneration enhancement rather than treeline shift. In eastern Tibet, an abrupt recruitment in the 1970s but no significant upward movement of treeline position was found in eastern Tibet smith fir treeline (
With increasing warming, the length of growing season is significantly extended. However, little effect of increasing growing season on tree ring growth. For example, in Sygera Mts eastern Tibet, a weather station record at timberline (4390 m) and modeled length of growing season has significantly extended by 21.2 days, with a significant delay of its end (by 14.6 days) rather than from an earlier onset (by 6.6 days). Nevertheless, lengthening of the growing season did not yet enhance radial growth of Smith firs at the timberline (
However, shrub species competition in the treeline ecotone may become a barricade to slow down upward movement of treeline (
In summary, the timberlines of Himalayan region are among the highest over the world due to great mass elevation effect of the Tibetan Plateau. Simultaneously this area also represents the most diverse timberline species and thus geomorphology and tree species diversity determine the abundant timberline growth forms and timberline forms. In contrast to air temperature isotherms of timberline, growing season mean soil temperature at the soil depth of 10 cm represents consent thermal conditions of nearly 7℃ over the world. It is indicated low temperature is the most dominant controlling factors of timberline. Trees at timberline ecotone are not in a physiological inferiority compared to other life forms. It appears that low temperature limits carbon sink rather than carbon source in timberline. So timberline is a threshold of growth limitation caused by low temperature. There is no reason to assume that water and nutrient are the key factors to determine timberline position, specifically they are at the most the modulating factors in local environment. In the future global warming scenario, timberline will be expected to advance to higher altitude, but in most cases, increasing tree recruitment and tree growth is the case rather timberline advancing.
References
[2] K Birmann, C Korner. Nitrogen status of conifer needles at the alpine treeline. Plant Ecology & Diversity, 2, 233-241(2009).
[3] S Chang D H. The vegetation zonation of the Tibetan Plateau. Mountain Research and Development, 1, 29-48(1981).
[4] G Hoch, C Körner. Growth, demography and carbon relations of
[5] K Holtmeier F, G Broll. Treeline advance — Driving processes and adverse factors. Landscape Online, 1, 1-32(2007).
[7] C Körner. Worldwide positions of alpine treelines and their causes. In: Beniston M, Inne J L (eds.). The impacts of climate variability on forests, lecture notes in earth sciences(1998).
[8] C Körner. Alpine plant life: Functional plant ecology of high mountain ecosystems (2nd edition). Berlin: Springer.(2003).
[9] C Körner. Climatic treelines: Conventions, global patterns, causes. Erdkunde, 61, 316-324(2007).
[10] C Körner. Alpine treelines — Functional ecology of the global high elevation tree limits. Basel: Springer.(2012).
[12] C Körner. Water, nutrient and carbon relations. In: Körner C (ed.). Alpine Treelines. Basel: Springer, 151-168(2012).
[13] C Körner. Alpine ecosystems and the high-elevation treeline. In: Sven Erik J, Brian D F (eds.). Encyclopedia of ecology, 407-413(2018).
[15] C Kuan. The geography of conifers in Sichuan(1982).
[16] M Li, W Xiao, P Shi et al. Nitrogen and carbon source-sink relationships in trees at the Himalayan treelines compared with lower elevations. Plant, Cell & Environment, 31, 1377-1387(2008).
[17] W Li, P Chou. The geographical distribution of the spruce-fir forest in China and its modelling. Mountain Research and Development, 4, 203-212(1984).
[18] W Li, Y Han, M Shen. Tibetan forests.(1985).
[19] E Liang, B Dawadi, N Pederson et al. Is the growth of birch at the upper timberline in the Himalayas limited by moisture or by temperature?. Ecology, 95, 2453-2465(2014).
[20] E Liang, C Leuschner, C Dulamsuren et al. Global warming-related tree growth decline and mortality on the North-Eastern Tibetan Plateau. Climatic Change, 134, 163-176(2016).
[23] B Liu, Y Li, D Eckstein et al. Has an extending growing season any effect on the radial growth of Smith fir at the timberline on the southeastern Tibetan Plateau?. Trees, 27, 441-446(2013).
[24] Z Liu. The vegetation of Gongga Mountain.(1985).
[25] G Liu Z, C Zhong Z. Sichuan vegetation(1980).
[26] G Liu Z, C Zhong Z. Sichuan vegetation(1980).
[27] G Miehe, S Miehe, J Vogel et al. Highest treeline in the northern hemisphere found in southern Tibet. Mountain Research and Development, 27, 169-173(2007).
[29] M Mou Y, O Fang, X Cheng et al. Recent tree growth decline unprecedented over the last four centuries in a Tibetan juniper forest. Journal of Forestry Research, 30, 1429-1436(2019).
[30] S Negi P. Climate change, alpine treeline dynamics and associated terminology: Focus on northwestern Indian Himalaya. Tropical Ecology, 53, 371-374(2012).
[31] S Panigrahy, D Anitha, M Kimothi M et al. Timberline change detection using topographic map and satellite imagery. Tropical Ecology, 51, 87-91(2010).
[32] M Rahman, M Islam, A Bräuning. Tree radial growth is projected to decline in South Asian moist forest trees under climate change. Global and Planetary Change, 170, 106-119(2018).
[33] S Rawal R, S Pangtey Y P. Distribution and structural-functional attributes of trees in the high altitude zone of Central Himalaya, India. Vegetatio, 112, 29-34(1994).
[34] S Rawat D. Monitoring ecosystem boundaries in the Himalaya through an “eye in the sky”. Current Science, 102, 1352-1354(2012).
[35] U Schickhoff. The upper timberline in the Himalayas, Hindu Kush and Karakorum. In: Broll G, Keplin B (eds.). A review of geographical and ecological aspects. mountain ecosystems. studies in treeline ecology, 275-354(2005).
[36] U Schweinfurth. The horizontal and vertical spread of vegetation in Himalayas. Bonn Geographical Treatises, 20, 373-379(1957).
[37] P Shi. A study on the vegetation ecology of subalpine timberline ecotone. PhD diss., Beijing: Chinese Academy of Sciences(1999).
[38] P Shi, C Körne, G Hoch. End of season carbon supply status of woody species near the treeline in western China. Basic and Applied Ecology, 7, 370-377(2006).
[39] P Shi, C Körner, G Hoch. A test of the growth-limitation theory for alpine tree line formation in evergreen and deciduous taxa of the eastern Himalayas. Functional Ecology, 22, 213-220(2008).
[43] P Singh C, S Panigrahy, A Thapliyal et al. Monitoring the alpine treeline shift in parts of the Indian Himalayas using remote sensing. Current Science, 102, 559-562(2012).
[44] A Stainton J D. Forest of Nepal. London: John Murray.(1972).
[45] C Stevens G, F Fox J. The causes of treeline. Annual Review of Ecosystem and Systematics, 22, 171-191(1991).
[46] W Tranquillini. Physiology ecology of the alpine timberline — Tree existence at high altitudes with special reference to the European Alps. Berlin: Springer(1979).
[47] W Tranquillini. Climate and physiology of trees in the alpine timberline regions. Palder Klimaforschung, 9, 127-136(1993).
[48] X Wang, L Zhang, Y Fang J. Geographical differences in alpine timberline and its climate interpretation in China. Acta Geographica Sinica, 59, 871-879(2004).
[49] Y Wang, J Julio Camarero, T Luo et al. Spatial patterns of Smith fir alpine treelines on the south-eastern Tibetan Plateau support that contingent local conditions drive recent treeline patterns. Plant Ecology & Diversity, 5, 311-321(2012).
[50] P Wardle. Alpine timberline. In: Ives J D, Barry R G (eds.). Arctic and alpine environments, 371-402(1974).
[53] Y Wu C. Vegetation of China.(1980).
[55] W Zhang J, T Wang J, L Chen W et al. Tibetan vegetation.(1988).
[56] D Zheng, Q Yang. Some problems on the altitudinal belts in southeastern Qinghai-Xizang (Tibetan) Plateau. Acta Geographica Sinica, 40, 60-69(1985).
[57] D Zheng, R Zhang, Q Yang. Physic-geographic differentiation of the Qinghai-Tibetan Plateau. In: Zheng D (ed.). Geological and ecological studies of Qinghai-Xizang Plateau, 1851-1860(1981).
[58] D Zheng, R Zhang, Y Yang Q. On physical zones in the Qinghai-Tibetan Plateau. Acta Geographica Sinica, 34, 1-11(1979).
[59] Y Zheng. A study on the correlation between montane forest vegetation and climate in the southeastern part of Qinghai-Tibetan Plateau. PhD diss., Beijing: Institute of Geography, Chinese Academy of Sciences(1995).
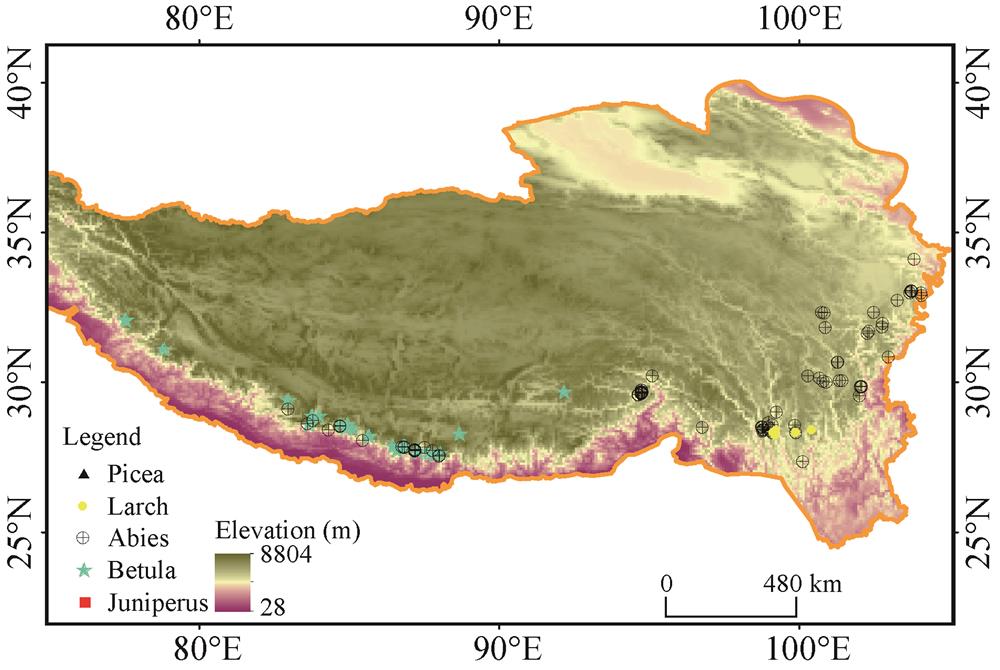
Set citation alerts for the article
Please enter your email address