Kunpeng Jia, Xiaohan Wang, Xinjie Lü, Ping Xu, Zhenlin Wang, Chee Wei Wong, Gang Zhao, Yan-Xiao Gong, Zhenda Xie, Shining Zhu, "Robust second-order correlation of twin parametric beams generated by amplified spontaneous parametric down-conversion," Chin. Opt. Lett. 18, 121902 (2020)

Search by keywords or author
- Chinese Optics Letters
- Vol. 18, Issue 12, 121902 (2020)
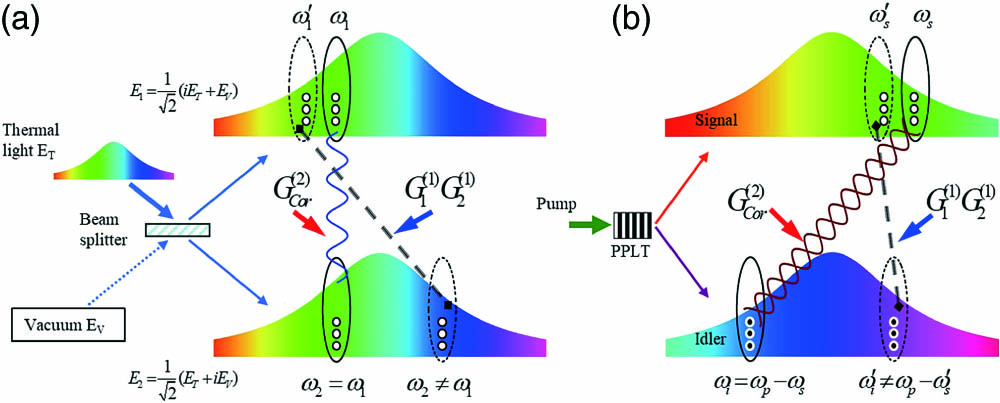
Fig. 1. Comparison of the second-order correlation of thermal light and ASPDC. (a) Thermal light correlation. The thermal light is mixed with vacuum field at a beam splitter. Therefore, the light fields and are from the same source even though is measured at different space–time points. can be expressed as , where the correlated term comes from the same frequency components in the two split beams, and the background term comes from different frequency components in the two split beams. (b) ASPDC correlation. The temporal correlation can be measured directly between the signal and idler beams, where the correlated term comes from signal frequency mode and its inherently correlated counterpart in idler mode , while the background term comes from the random combination with and .
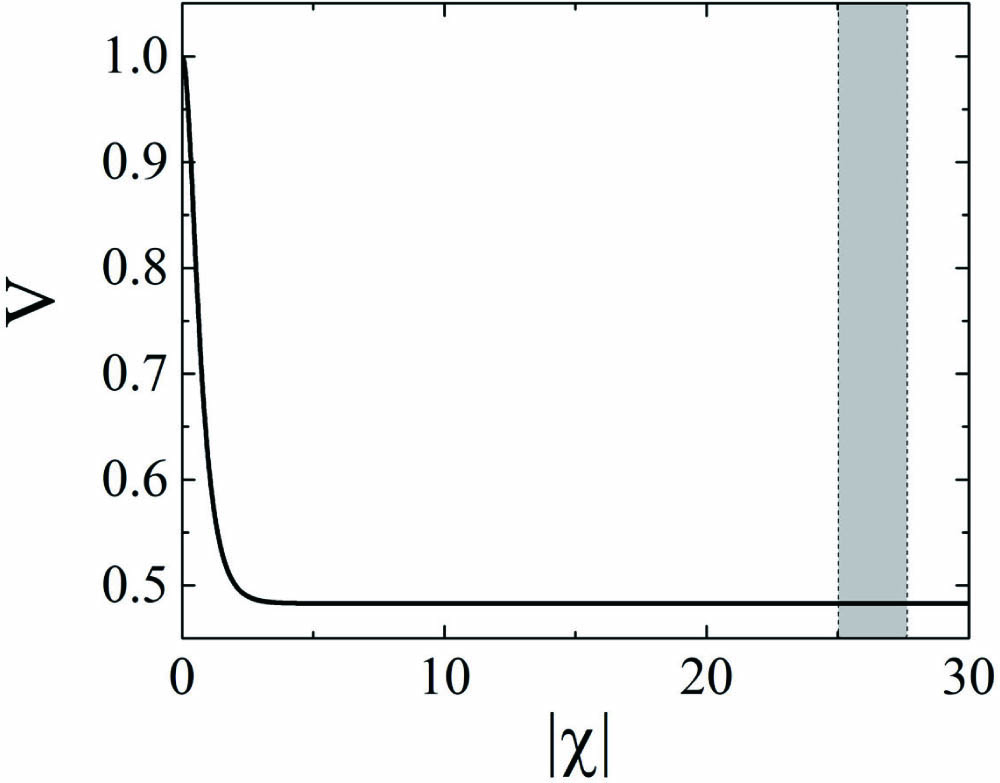
Fig. 2. Simulated visibility as a function of gain parameter. The visibility drops from one to about 48.3%, as the parametric process transforms from SPDC to ASPDC. The two vertical bars mark the range of the gain level achieved in our experiment, where the visibility can be considered as constant.
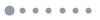
Fig. 3. Schematic of experiment setup. BS, beam splitter; DM, dichromatic mirror; IF, interference filter; FFPC, fiber Fabry–Perot cavity; FPC, fiber polarization controller; FBG, fiber Bragg grating; and , fiber connectors; VOA, variable optical attenuator; HPF, 600 MHz high-pass RF filter (removable).
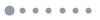
Fig. 4. ASPDC energy as a function of pump pulse energy.
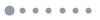
Fig. 5. (a) Correlation of twin beams as a function of relative time delay, where the red curve shows the background given by the pulse profile of the twin beams. (b) Normalized correlation with exponential decay fit. (c) Visibility as a function of pump pulse energy and transmission of variable optical attenuator (VOA), respectively. (d) Correlation measurement with 600 MHz high-pass RF filters in the photon current.
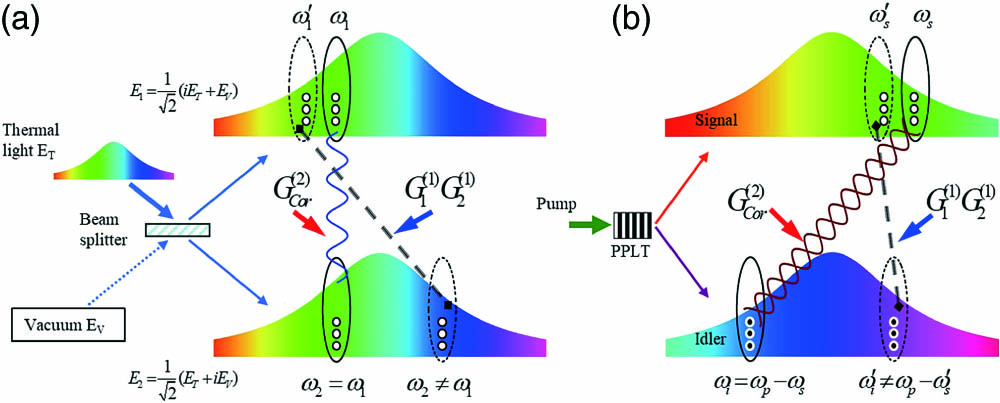
Set citation alerts for the article
Please enter your email address