F. Bisesto, M. Galletti, M. P. Anania, M. Ferrario, R. Pompili, M. Botton, A. Zigler, F. Consoli, M. Salvadori, P. Andreoli, C. Verona, "Single-shot electrons and protons time-resolved detection from high-intensity laser–solid matter interactions at SPARC_LAB," High Power Laser Sci. Eng. 7, 03000e53 (2019)

Search by keywords or author
- High Power Laser Science and Engineering
- Vol. 7, Issue 3, 03000e53 (2019)
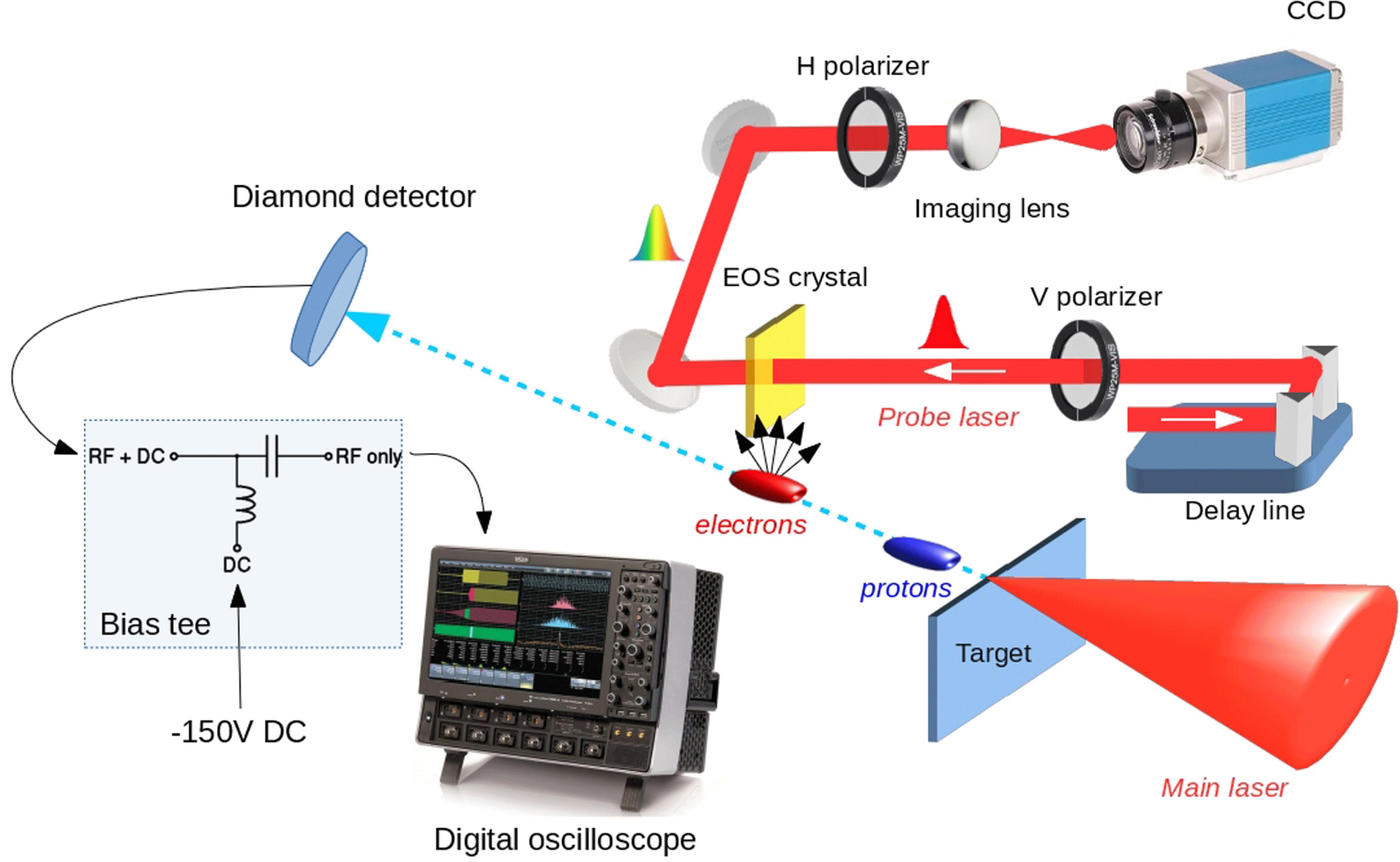
Fig. 1. Experimental setup. The FLAME laser is sent to a stainless steel target. The charged particles emitted during this interaction are revealed by two single-shot time-resolved measurements: an electro-optical sampling diagnostic, able to measure the electric field carried by relativistic fast electrons, and a time-of-flight diamond detector, used to measure the temporal distribution of protons arriving on it and retrieve their energy spectra.
![Time-of-flight detector geometry. Schematic representation of the device layer structure (left) and picture of the surface Al interdigitated electrodes (right). The metal fingers were processed to $20~\unicode[STIX]{x03BC}\text{m}$ in width, with a spacing between the electrodes of $20~\unicode[STIX]{x03BC}\text{m}$. The detector active area was approximately $2~\text{mm}^{2}$.](/richHtml/hpl/2019/7/3/03000e53/img_2.gif)
Fig. 2. Time-of-flight detector geometry. Schematic representation of the device layer structure (left) and picture of the surface Al interdigitated electrodes (right). The metal fingers were processed to $20~\unicode[STIX]{x03BC}\text{m}$ in width, with a spacing between the electrodes of $20~\unicode[STIX]{x03BC}\text{m}$ . The detector active area was approximately $2~\text{mm}^{2}$ .
![Typical 2D electric field carried by fast electrons as seen by our EOS diagnostic tool. The signal thickness is related to the temporal duration of the electric field. The typical shape is a direct consequence of our setup geometry[27].](/Images/icon/loading.gif)
Fig. 3. Typical 2D electric field carried by fast electrons as seen by our EOS diagnostic tool. The signal thickness is related to the temporal duration of the electric field. The typical shape is a direct consequence of our setup geometry[27].
![Line profile traced along the signal in Figure 3. The measured electric field shows a peak value $E_{0}=1.5~\text{MV}/\text{m}$ and a temporal duration $\unicode[STIX]{x1D70F}=0.5$ ps FWHM. The fast electron charge also has been retrieved with a value of $Q=6$ nC.](/Images/icon/loading.gif)
Fig. 4. Line profile traced along the signal in Figure 3 . The measured electric field shows a peak value $E_{0}=1.5~\text{MV}/\text{m}$ and a temporal duration $\unicode[STIX]{x1D70F}=0.5$ ps FWHM. The fast electron charge also has been retrieved with a value of $Q=6$ nC.
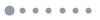
Fig. 5. Typical signal provided by the TOF detector as seen by our 2 GHz Lecroy 620ZI oscilloscope. As one can see, it is possible to distinguish between two different signals arriving at different times: the first is associated with X-rays and low-energy electrons coming at the early stage of the interaction; the second is related to protons accelerated through the TNSA mechanism.
![Monte Carlo simulations by the SRIM code results for $10~\unicode[STIX]{x03BC}\text{m}$ of aluminium.](/Images/icon/loading.gif)
Fig. 6. Monte Carlo simulations by the SRIM code results for $10~\unicode[STIX]{x03BC}\text{m}$ of aluminium.
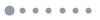
Fig. 7. Proton energy spectra retrieved from the data in Figure 5 with (black line) and without (red line) taking into account the aluminium filter. Tolerances present in the black curve are only due to the energy spread of the incoming proton beam caused by crossing the foil.
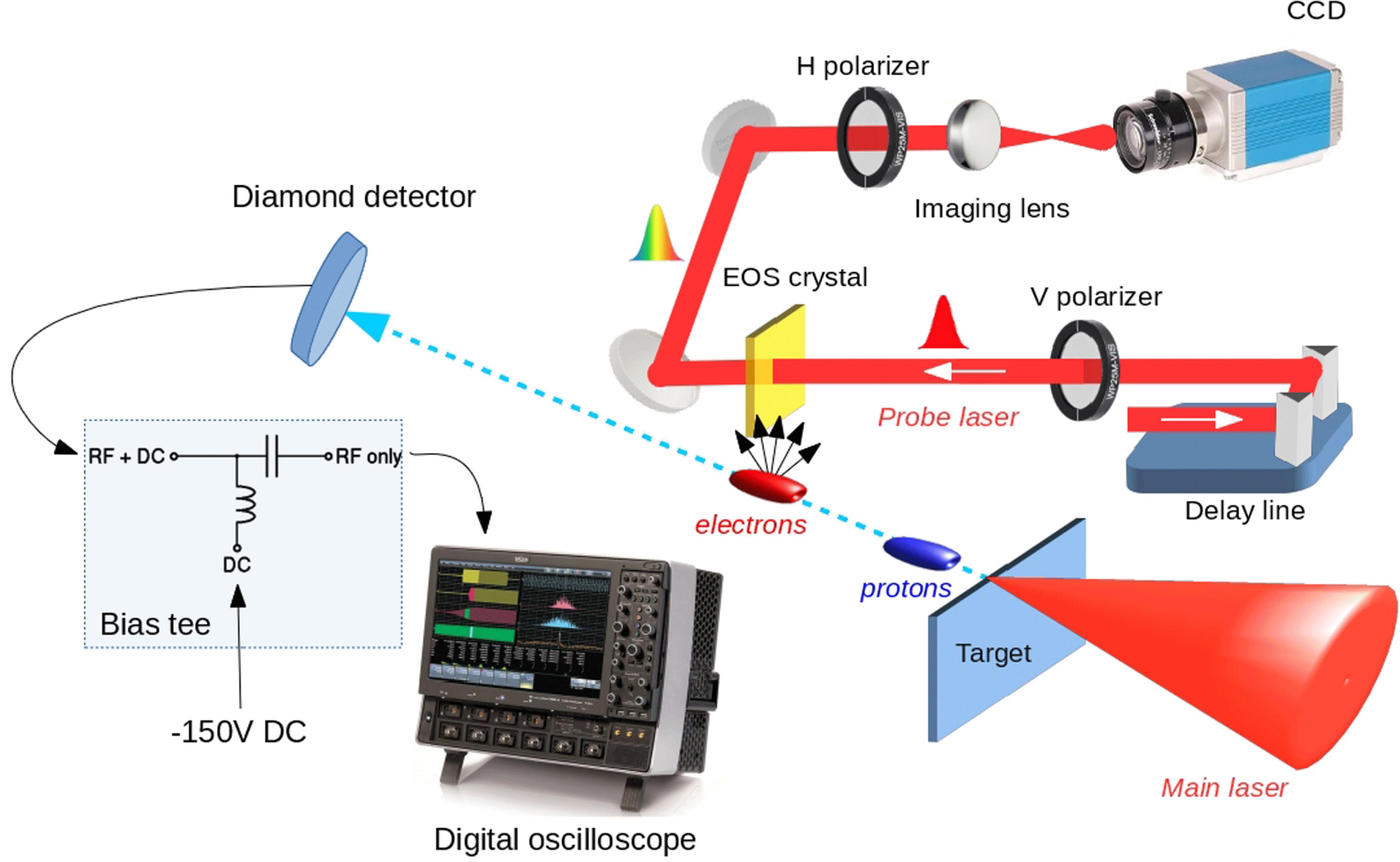
Set citation alerts for the article
Please enter your email address