
- Chinese Optics Letters
- Vol. 21, Issue 3, 031406 (2023)
Abstract
1. Introduction
Obtaining a high peak output power from a CW diode-pumped solid-state laser is important for applications such as nonlinear optics, high-precision material processing, Raman spectroscopy, and other uses. In Refs. [1–3], a method was proposed that allows using one traveling wave acousto-optic modulator (AOM) and an end spherical mirror (SM) of the cavity (SMAOM method) to simultaneously obtain mode locking and
In a laser with the SMAOM method, mode locking occurs due to the return of one of the beams diffracted by the AOM back into the laser cavity. Returning to the cavity, this beam receives a frequency addition equal to twice the ultrasonic wave frequency
In the present work, mode locking by a second-order subharmonic in a diode-pumped Nd:YAG laser with the SMAOM method is studied. Mode locking is achieved by returning a diffracted beam to the resonator; the frequency of such a beam is shifted by half the intermode interval of the resonator using an acousto-optic traveling wave modulator. The dynamics of laser generation is studied, and laser generation is obtained in the QML and autoQML modes. Also, the results on the use of this laser for obtaining ultrashort pulses with a tunable frequency in the near-IR, mid-IR and in the visible range are presented.
Sign up for Chinese Optics Letters TOC. Get the latest issue of Chinese Optics Letters delivered right to you!Sign up now
2. Experiment Setup
The experimental scheme of the laser is shown in Fig. 1. The active element was a Nd:YAG crystal 2 mm in diameter and 65 mm in length with diode side pumping at a wavelength of 808 nm. The radii of curvature of the spherical mirrors M1 and M2 were 0.5 and 0.3 m, respectively, and their reflection coefficients at the operating wavelength
Figure 1.Laser circuit. M1 and M2, cavity mirrors; AOM, acousto-optic modulator; Nd:YAG, active element; 0, zeroth-order diffraction beam; 1, first-order diffraction beam.
3. Experimental Results
As in previous works[3,16,17], the resonator length was tuned when a continuous ultrasonic frequency signal was applied to the modulator by moving one of the resonator mirrors to achieve stable mode locking in continuous mode (CWML). The diffraction efficiency of the AOM was determined by measuring the power of zeroth-order and first-order diffraction beams emerging from the resonator through mirror M2 and was
Figure 2.Oscillograms of the output laser radiation at kd ≈ 7%. (a) ΔL = 0, (b) ΔL = 80 µm.
The QML generation mode was carried out at
Figure 3.Oscillogram of a lasing pulse at a wavelength of 1.064 µm in QML mode. The division along the abscissa axis is 20 ns.
The pulse duration was measured using an optical intensity autocorrelator, assembled according to a noncollinear phase-matching scheme in a KTP crystal with a thickness of 200 µm. The second-harmonic signal was recorded by a photodiode with slow response time, which ensured the averaging of the output signal of the autocorrelator. The measurement result is shown in Fig. 4. The form of the autocorrelation function corresponded to the form of the Lorentz function. The pulse duration was
Figure 4.Autocorrelation function in QML generation mode.
A series of preliminary experiments on mode locking in this laser using subharmonics of the third and fourth orders were carried out. This was realized by shortening the resonator length to 50 cm and 37.5 cm, respectively, while the AOM still operated at f = 50,049.6 kHz. In both cases, complete mode locking and laser generation in the QML regime were obtained, but the output characteristics have not yet been measured.
4. Cascade Conversion of Laser Radiation to the Region of 620 nm
The radiation shown in the Fig. 1 laser in the QML mode with a train frequency of 5 kHz was used to pump a superluminescent parametric generator and then convert its frequency to the visible region of the spectrum [cascade mixing of its signal wave (1475 nm) with a pump wave (1064 nm) for obtaining tunable radiation in the 620 nm region]. Figure 5 shows a diagram of the experimental setup. The characteristics of this scheme related to obtaining superluminescent parametric generation on a lithium niobate crystal with a periodic domain structure (PPLN) are described in detail in Ref. [18]. In this work, the same experimental results were obtained: the conversion efficiency was 83% for absorbed pump power.
Figure 5.Schematic of a setup for obtaining superluminescent generation tunable in the 620 nm region. 1, Nd:YAG pump laser; 2, collimator; 3, focusing lens; 4, PPLN crystal (50 mm length, polarization period 29.5 µm, ee-e phase synchronism); 5, selective mirror (HR at 3200-3850 nm, HT at 1050–1700 nm); 6, focusing lens; 7, LBO crystal (15 mm length, Θ = 90°, φ = 0°, ee-o synchronism); 8, prism (TF10); 9, diaphragm; 10 and 11, measuring equipment.
The diameter of the pump radiation waist in the PPLN crystal was 160 µm. At a power incident on the PPLN crystal of 420 mW, the OPG output power was
The average radiation power at a wavelength of 620 nm was 5 mW. The pulse duration was measured using a first-order autocorrelation function, which is the dependence of the visibility of the interference fringes on the difference in the path of the rays in the Michelson interferometer and gives the same results as nonlinear measurements[19]. The pulse duration was
5. Conclusions
A diode-pumped Nd:YAG laser with the SMAOM method of mode locking, operating in the mode-locked and
References
[1] V. I. Donin, D. V. Yakovin, A. V. Gribanov. Q-switching and mode-locking in a diode-pumped frequency-doubled Nd: YAG laser. Quantum Electron., 42, 107(2012).
[2] V. I. Donin, D. V. Yakovin, A. V. Gribanov, M. D. Yakovin. New method of Q-switching with mode locking in solid-state lasers. J. Opt. Technol., 85, 193(2018).
[3] A. V. Gribanov, V. I. Donin, D. V. Yakovin. Generation regimes of an Nd: YAG laser with mode locking by a travelling-wave acousto-optic modulator and spherical mirror. Quantum Electron., 48, 699(2018).
[4] V. I. Donin, D. V. Yakovin, A. V. Gribanov. Self-organization of the Q-switched mode-locked regime in a diode-pumped Nd: YAG laser. JETP Lett., 101, 783(2015).
[5] O. E. Nanii, A. I. Odintsov, A. I. Panakov, A. P. Smirnov, A. I. Fedoseev. QML-generation dynamics of a solid-state laser with an acousto-optic travelling wave modulator. Quantum Electron., 47, 1000(2017).
[6] O. E. Nanii, A. I. Odintsov, A. I. Panakov, A. P. Smirnov, A. I. Fedoseev. Simultaneous mode locking and Q-switching in a solid-state laser with a travelling-wave acousto-optic modulator and retroreflector. Quantum Electron., 49, 119(2019).
[7] A. A. Apolonskiĭ, V. I. Donin, T. T. Timofeev, D. A. Shapiro. Mode locking in an ion laser at multiple frequencies. Quantum Electron., 16, 76(1986).
[8] Y. M. Chang, J. Lee, J. H. Lee. A Q-switched, mode-locked fiber laser employing subharmonic cavity modulation. Opt. Express, 19, 26627(2011).
[9] I. Hong, K. Lee, J. H. Lee. 532-nm second harmonic generation with enhanced efficiency using subharmonic cavity modulation-based quasi-Q-switched-mode-locked pulses. Opt. Express, 28, 25431(2020).
[10] S. Arahira, Y. Ogawa. Synchronous mode-locking in passively mode-locked semiconductor laser diodes using optical short pulses repeated at subharmonics of the cavity round-trip frequency. IEEE Photon. Technol. Lett., 8, 191(1996).
[11] A. Nirmalathas, H. F. Liu, Z. Ahmed, D. Novak, Y. Ogawa. Subharmonic synchronous and hybrid mode-locking of a monolithic DBR laser operating at millimeter-wave frequencies. IEEE Photon. Technol. Lett., 9, 434(1997).
[12] C. R. Poole. Subharmonic injection locking phenomena in synchronous oscillators. Electron. Lett., 26, 1748(1990).
[13] H. G. Oltman, C. H. Nonnemaker. Subharmonically injection phase-locked Gunn oscillator experiments. IEEE Trans. Microw. Theory Techn., 17, 728(1969).
[14] C. H. Chien, G. C. Dalman. Subharmonically injected phase-locked IMPATT-oscillator experiments. Electron. Lett., 6, 240(1970).
[15] H. T. Huang, J. L. He, X. W. Fan, Y. Zhong, H. L. Chai, Y. Wang. The acousto-optic Q-switched mode-locking Nd:YVO4 laser. Opt. Laser Technol., 40, 828(2008).
[16] A. V. Gribanov, D. V. Yakovin, M. D. Yakovin. Regimes of pulsed generation in a Nd:YAG-laser with different optical circuits of resonators. Optoelectron. Instrum. Data Process., 56, 310(2020).
[17] A. V. Gribanov, D. V. Yakovin, M. D. Yakovin. Nd:YAG laser with mode locking using a travelling-wave acousto-optic modulator and spherical mirror. J. Opt. Technol., 87, 326(2020).
[18] V. I. Donin, D. V. Yakovin, M. D. Yakovin, A. V. Gribanov. Superluminescent high-efficient parametric generation in PPLN crystal with pumping by a Q-switched mode locked Nd:YAG laser. Laser Phys. Lett., 15, 035005(2018).
[19] R. L. Smith, C. O. Alley. A linear technique for the measurement of ultrashort optical pulse widths. Opt. Commun., 1, 262(1970).
[20] M. F. Becker, D. J. Kuizenga, D. W. Phillion, A. E. Siegman. Analytic expressions for ultrashort pulse generation in mode-locked optical parametric oscillators. J. Appl. Phys., 45, 3996(1974).
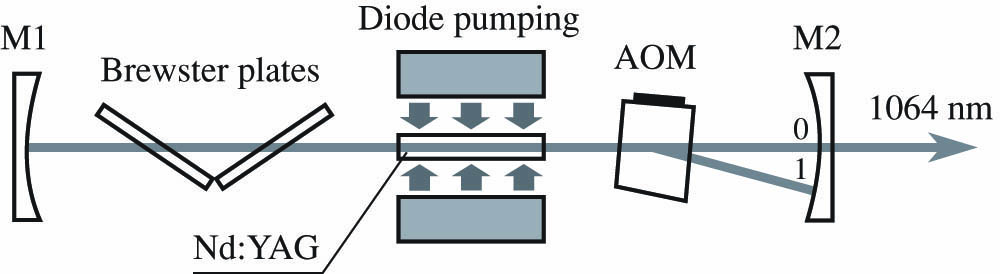
Set citation alerts for the article
Please enter your email address