
- Chinese Optics Letters
- Vol. 21, Issue 2, 021405 (2023)
Abstract
1. Introduction
The optical vortex generally refers to the Laguerre–Gaussian (
Nowadays, generation and modulation of OAM in the laser cavities have been intensively studied with different techniques, such as spot-defect mirror[14], annular-shape beam pumping[15,16], and intracavity spiral phase plate[17]. However, wavelength tuning of vortex beams with well-maintained spatial amplitude and phase structure is scarcely reported from a laser cavity. To date, the reflective volume Bragg grating (VBG) has been theoretically and experimentally proved to be an effective wavelength selector for optical vortices with well-conserved OAM[18]. Most recently, a birefringent filter (BF) was experimentally used in a Yb:CaGdAlO4 (Yb:CALGO) vortex laser and exhibited similar wavelength tuning ability for vortex beams[19]. Unfortunately, all of the previous reports have been focused on the near-infrared (IR) spectral region, and the wavelength tuning range was less than 50 nm limited by the gain spectrum of laser materials or cavity design[18,19]. So, wide tunability of the vortex laser, in particular, in the mid-IR region is desired from the viewpoint of their potential applications.
The thulium-doped yttrium aluminum perovskite (Tm:YAP) crystal has a high quantum efficiency for laser operation at 2 µm due to the existence of a self-quenching mechanism between the
Sign up for Chinese Optics Letters TOC. Get the latest issue of Chinese Optics Letters delivered right to you!Sign up now
2. Cavity Design and Experimental Layout
Generation of a vortex laser in this work was realized by using an annular pumping technique, which is, in principle, based on a selection of the transverse mode in the cavity. The BF is a 2-mm-thick octagonal quartz plate (a uniaxial crystal with refractive index of
Figure 1.(a) Calculated effective pumping areas versus cavity length for different LG modes with an annular pump. (b) Schematic of the 2 µm wavelength-tunable Tm:YAP vortex laser. LD, laser diode; L, lens; IM, input mirror; BF, birefringent filter; OC, output coupler.
Figure 1(b) shows the schematic of the wavelength tuning vortex laser. The pump source (LD) was a fiber (100 µm core diameter and 0.22 NA) coupled LD with a center wavelength of 793 nm. The pump light was at first collimated by a plano-convex lens (
3. Results and Discussions
For comparison, we at first studied the tuning performance of the fundamental mode by adjusting the cavity to a length of
Figure 2.(a) Laser slope efficiency, (b) wavelength tuning performance, and (c) the polarization measurement of the annular light pumped Tm:YAP laser with E∥b and E∥c polarization. Inset in (a): beam pattern and the corresponding optical spectrum of the Tm:YAP laser without BF.
Following the theoretical analysis, wavelength tuning of the optical vortices (
Figure 3.Simulated beam radius of laser in the YAP crystal as a function of absorbed pump power by considering the thermal lens effect. Inset: beam profiles of the output laser at different pump powers.
Next, we studied the wavelength tuning performance of the vortex laser for
Figure 4.(a) Laser output performance of the LG0,+1 modes with and without the BF. (b) Beam profiles and the corresponding interference fringe patterns of LG0,+1 and LG0,-1 modes. Insets in (a): optical spectra and polarization measurement of LG0,+1 and LG0,-1 modes without the BF in the cavity.
For the
Figure 5.(a) Measured tuning spectra for LG0,+1 and LG0,-1 modes at the fixed absorbed pump power of 4.27 W. (b) and (c) The measured spectral lines at several typical wavelengths for the LG0,+1 and LG0,-1 modes.
4. Conclusion
In summary, we reported on the first, to the best of our knowledge, wavelength-tunable vortex laser in the 2 µm spectral range by employing a BF. A continuous tuning range of 76 nm from 1920.4 to 1996.6 nm has been demonstrated for the
References
[1] L. Allen, M. W. Beijersbergen, R. J. C. Spreeuw, J. P. Woerdman. Orbital angular momentum of light and the transformation of Laguerre-Gaussian laser modes. Phys. Rev. A, 45, 8185(1992).
[2] J. Wang, J. Y. Yang, I. M. Fazal, N. Ahmed, Y. Yan, H. Huang, Y. Ren, Y. Yue, S. Dolinar, M. Tur. Terabit free-space data transmission employing orbital angular momentum multiplexing. Nat. Photonics, 6, 488(2012).
[3] D. G. Grier. A revolution in optical manipulation. Nature, 424, 810(2003).
[4] M. P. J. Lavery, F. C. Speirits, S. M. Barnett, M. J. Padgett. Detection of a spinning object using light’s orbital angular momentum. Science, 341, 537(2013).
[5] M. W. Beijersbergen, L. Allen, H. van der Veen, J. P. Woerdman. Astigmatic laser mode converters and transfer of orbital angular momentum. Opt. Commun., 96, 123(1993).
[6] M. W. Beijersbergen, R. P. C. Coerwinkel, M. Kristensen, J. P. Woerdman. Helical-wavefront laser beams produced with a spiral phase plate. Opt. Commun., 112, 321(1994).
[7] V. Y. Bazhenov, M. V. Vasnetsov, M. S. Soskin. Laser-beams with screw dislocations in their wave-fronts. JETP Lett., 52, 429(1990).
[8] N. Matsumoto, T. Ando, T. Inoue, Y. Ohtake, N. Fukuchi, T. Hara. Generation of high-quality higher-order Laguerre–Gaussian beams using liquid-crystal-on-silicon spatial light modulators. J. Opt. Soc. Am. A, 25, 1642(2008).
[9] D. Lin, J. M. O. Daniel, W. A. Clarkson. Controlling the handedness of directly excited Laguerre-Gaussian modes in a solid-state laser. Opt. Lett., 39, 3903(2014).
[10] D. J. Kim, J. W. Kim. Direct generation of an optical vortex beam in a single-frequency Nd:YVO4 laser. Opt. Lett., 40, 399(2015).
[11] D. Lin, W. A. Clarkson. Polarization-dependent transverse mode selection in an Yb-doped fiber laser. Opt. Lett., 40, 498(2015).
[12] J. L. Lu, H. F. Lin, G. Zhang, B. X. Li, L. Z. Zhang, Z. B. Lin, Y. F. Chen, V. Petrov, W. D. Chen. Direct generation of an optical vortex beam from a diode-pumped Yb:MgWO4 laser. Laser Phys. Lett., 14, 085807(2017).
[13] C. Y. Fu, Y. P. Lan. Dynamics of the Laguerre Gaussian TEM*0,l mode in a solid-state laser. Phys. Rev. A, 63, 063807(2001).
[14] A. Ito, Y. Kozawa, S. Sato. Generation of hollow scalar and vector beams using a spot-defect mirror. J. Opt. Soc. Am. A, 27, 2072(2010).
[15] Y. Chen, Y. Lan. Dynamics of the Laguerre Gaussian TEM01 mode in a solid-state laser. Phys. Rev. A, 63, 063807(2001).
[16] Y. Zhao, Z. Wang, H. Yu, S. Zhang, H. Zhang, X. Xu, J. Xu, X. Xu, J. Wang. Direct generation of optical vortex pulses. Appl. Phys. Lett., 101, 031113(2012).
[17] A. Ishaaya, N. Davidson, A. Friesem. Very high-order pure Laguerre-Gaussian mode selection in a passive Q-switched Nd:YAG laser. Opt. Express, 13, 4952(2005).
[18] Q. Liu, Y. Zhao, M. Ding, W. Yao, X. Fan, D. Shen. Wavelength- and OAM-tunable vortex laser with a reflective volume Bragg grating. Opt. Express, 25, 23312(2017).
[19] L. Zhao, Y. Yuan, L. Tong, F. Cai, W. Zhang, Y. Cai. Broadly tunable optical vortex beam in a diode-pumped Yb:CALGO laser. Opt. Laser Technol., 141, 107134(2021).
[20] R. C. Stoneman, L. Esterowitz. Efficient 1.94-pm Tm:YALO laser. IEEE J. Sel. Top Quantum Electron., 1, 78(1995).
[21] I. F. Elder, J. Payne. Diode-pumped, room-temperature Tm:YAP laser. Appl. Opt., 36, 8606(1998).
[22] G. Ghosh. Dispersion-equation coefficients for the refractive index and birefringence of calcite and quartz crystals. Opt. Commun., 163, 95(1999).
[23] X. L. Wang, J. Q. Yao. Transmitted and tuning characteristics of birefringent filters. Appl. Opt., 31, 4505(1992).
[24] N. Zhang, Z. X. Wang, S. D. Liu, W. Jing, H. Huang, Z. X. Huang, K. Z. Tian, Z. Y. Yang, Y. G. Zhao, U. Griebner, V. Petrov, W. D. Chen. Watt-level femtosecond Tm-doped “mixed” sesquioxide ceramic laser in-band pumped by a Raman fiber laser at 1627 nm. Opt. Express, 30, 23978(2022).
[25] J. W. Kim, W. A. Clarkson. Selective generation of Laguerre-Gaussian (LG0n) mode output in a diode-laser pumped Nd:YAG laser. Opt. Commun., 296, 109(2013).
[26] J. Körner, T. Lühder, J. Reiter, I. Uschmann, H. Marschner, V. Jambunathan, A. Lucianetti, T. Mocek, J. Hein, M. C. Kaluza. Spectroscopic investigations of thulium doped YAG and YAP crystals between 77 K and 300 K for short-wavelength infrared lasers. J. Lumin., 202, 427(2018).
[27] Y. Chen, M. M. Ding, J. L. Wang, L. Wang, Q. Y. Liu, Y. G. Zhao, Y. Liu, D. Shen, Z. P. Wang, X. G. Xu, V. Petrov. High-energy 2 µm pulsed vortex beam excitation from a Q-switched Tm:LuYAG laser. Opt. Lett., 45, 722(2020).
[28] M. M. Ding, Y. Chen, J. Wang, D. L. Yin, Y. Wang, P. Liu, Y. G. Zhao, D. Y. Tang, D. Y. Shen, Y. Liu, Z. P. Wang, X. G. Xu, V. Petrov. 2.7 µm optical vortex beam directly generated from an Er:Y2O3 ceramic laser. Opt. Lett., 44, 4973(2019).
[29] D. Lin, W. A. Clarkson. End-pumped Nd:YVO4 laser with reduced thermal lensing via the use of a ring-shaped pump beam. Opt. Lett., 42, 2910(2017).
[30] W. D. Zhang, K. Y. Wei, L. G. Huang, D. Mao, B. Q. Jiang, F. Gao, G. Q. Zhang, T. Mei, J. L. Zhao. Optical vortex generation with wavelength tunability based on an acoustically-induced fiber grating. Opt. Express, 24, 19278(2016).
[31] V. S. Lyubopytov, A. P. Porfirew, S. O. Gurbatov, S. Paul, M. F. Schumann, J. Cesar, M. Malekizandi, M. T. Haidar, M. Wegener, A. Chipouline, F. Küppers. Simultaneous wavelength and orbital angular momentum demultiplexing using tunable MEMS-based Fabry-Perot filter. Opt. Express, 25, 9634(2017).
[32] Q. Y. Liu, Y. G. Zhao, M. M. Ding, W. C. Yao, D. Y. Shen. Wavelength- and OAM-tunable vortex laser with a reflective volume Bragg grating. Opt. Express, 25, 23312(2017).
[33] S. Yao, G. Ren, Y. Shen, Y. Jiang, S. Jian. Tunable orbital angular momentum generation using all-fiber fused coupler. IEEE Photon. Technol. Lett., 30, 99(2018).
[34] M. Gong, F. Xing, M. Yuan. Wavelength-tunable Hermite–Gaussian modes and an orbital-angular-momentum-tunable vortex beam in a dual-off-axis pumped Yb:CALGO laser. Opt. Lett., 43, 291(2018).
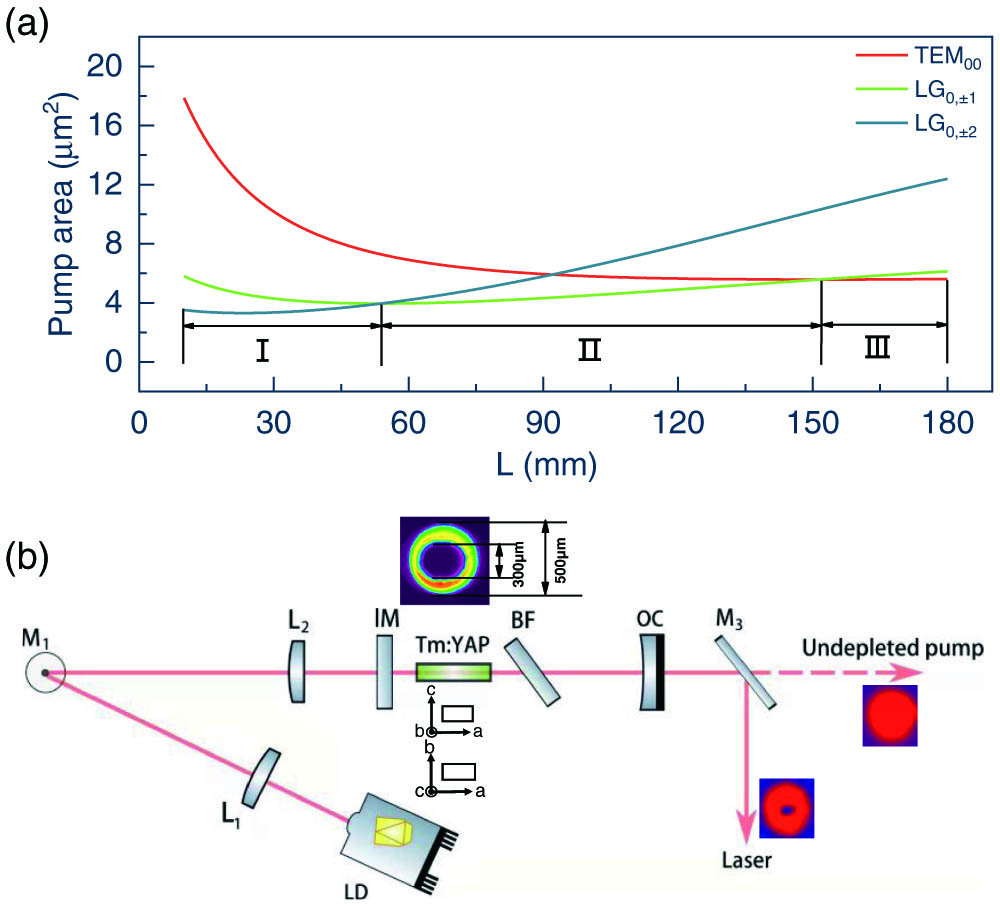
Set citation alerts for the article
Please enter your email address