Author Affiliations
1Key Laboratory of Semiconductor Materials Science, Beijing Key Laboratory of Low Dimensional Semiconductor Materials and Devices, Institute of Semiconductors, Chinese Academy of Sciences, Beijing 100083, China2Center of Materials Science and Optoelectronics Engineering, University of Chinese Academy of Sciences, Beijing 100049, China3Fachgebiet Angewandte Nanophysik, Institut für Physik & IMN MacroNano, Technische Universität Ilmenau, Ilmenau 98693, Germanyshow less
Since the long-lived charge has been demonstrated to be excited by light irradiation in the absence of the sacrificial reagent, the great promise can be articulated in two ways. On the one hand, it takes a significant step towards non-sacrificial reagent organic NPs photocatalysis. On the other hand, the long-lived charge opens up available applications for driving kinetically slow catalysis processes such as water oxidation. From a long-term perspective, the stability of organic semiconductor photocatalysts and the firmness of their binding will also be the research focus. Organic semiconductors present weak chemical bonds, which is detrimental for long-term photocatalysis, and the construction of heterostructures (for example, the D/A heterojunction of this work) or nano-assembly will be feasible strategies[13]. The weak non-covalent interactions between molecules, such as hydrogen bonding, dipolarization, π–π stacking, van der Waals forces, hydrophobic interactions, electrostatic interactions, and metal-ligand interactions, will be converted from inferiority for the advantages during the self-assembly process[14,15]. The challenge lies in achieving regular crystallization and smooth charge transport through perfect molecular packing. Furthermore, positioning catalytic active sites is increasingly attracting broad interest. Particularly, the complex molecular architectures and the ubiquitous bonds caused by diverse positions of the bonding molecules make it challenging to investigate the active sites for organic photocatalysts[16-18]. Ingenious design to embed synergistic Brønsted acid/base sites or active metal atoms into organic frameworks seems to reduce the difficulty of exploring active sites while remarkably enhancing catalysis[19].
There is no doubt that these results are charming and encouraging. The innovation and breakthroughs of this work are reflected in the HER performance and photophysical mechanism. This work announces the broadest wavelength range up to 900 nm and comparable EQEs to plasmonic photocatalyst systems for an organic photocatalyst to date, which highlights the potential of D/A organic heterojunction NPs for broad-spectrum HER, making these organic photocatalysts more ready for use in real applications. Careful consideration and ingenious comparisons are carried out throughout the whole photophysical exploring process, and the results fill the gap in the field of organic photocatalysis. In conclusion, this work provides an in-depth understanding of the photophysics in organic D/A heterojunction photocatalysts. Particularly, this thinking is conducive to further directing the research focus to the intrinsic mechanisms of the photocatalytic processes, which may be the key to the current breakthrough in solar energy conversion efficiency.
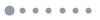
Figure 1.(Color online) (a) Bright-field cryo-TEM images of intermixed PM6:Y6 7 : 3 NPs and (b) phase-separated core-shell PM6:PCBM 2 : 8 NPs. (c) Energy level diagram of PM6, Y6, and PCBM measured by UPS and IPES. The dashed lines correspond to the proton reduction potential (H+/H2), water oxidation potential (O2/H2O), and the calculated potential of the two-hole oxidation of ascorbic acid to dehydroascorbic acid in solution (DHA/AA) at pH 2 (the experimentally measured pH of 0.2 mol/L ascorbic acid).
To address the challenge in EQE, one strategy is to configure donor/acceptor (D/A) organic heterojunction to obtain excellent charge separation. In a recent article (Nat. Energy, 2022, 7, 340)[11], Kosco and colleagues constructed two kinds of D/A organic heterojunction for hydrogen evolution reaction (HER) containing a typical donor polymer PBDB-T-2F (PM6) and two common acceptors named non-fullerene BTP-4F (Y6) and fullerene PCBM with the optimized D/A ratios. As shown inFigs. 1(a) and1(b), the authors developed blends with different morphologies of intermixed and phase-separated states due to the different miscibility via the mini-emulsion method. There is an energy level matching reflecting not only in the energy levels of the donor and acceptor but also in the alignment relationship with the reduction potential of water and the oxidation potential of the sacrificial agent (Fig. 1(c)). Impressively, photophysics is also given adequate attention in this work. A variety of characterization methods containing ultrafast transient absorption spectroscopy (uf-TAS) and photoinduced absorption spectroscopy (PIAS) were carried out to explore photophysical steps closely related to semiconductor photocatalysis (Fig. 2), such as charge generation, exciton dissociation, and charge transfer[12]. It is revealed that the second-scale long-life charges are induced only by photoexcitation in the nanoparticle-formed D/A heterojunction whether in the presence or absence of Pt and sacrificial agent. It was found that no measurable long-lived charge accumulation was observed from the spin-coated PM6:PCBM films. This is noteworthy and highlights the unique advantage of nanoscale D/A heterojunctions. Their results showed that organic D/A heterojunction NPs can inherently generate long-lived reactive charges, thereby allowing them to exhibit efficient photocatalytic HER performance.
The urgent need to replace conventional fossil fuels with clean energy has stimulated a large number of research efforts on photocatalytic hydrogen evolution[1-4]. Alternatively, organic semiconductors with tunable light absorption, well-positioned band edges, and excellent charge separation are highly expected[5-8]. Conventionally, a semiconductor material with a wide band gap has a larger exciton binding energy, while a semiconductor material with a narrow band gap has a smaller exciton binding energy[9]. Since smaller exciton binding energies are favorable for exciton separation, choosing a semiconductor with a suitable bandgap seems to be the first step toward high solar-to-hydrogen efficiency. The tunable light-harvesting ability determines the advantage and potential of organic semiconductors as photocatalysts. However, the insufficient external quantum efficiency (EQE) and the underlying photophysical mechanism remain restricting the orientation toward industrialization[10].
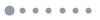
Figure 2.(Color online) Ultrafast TAS characterization for neat PM6, PM6:Y6 7 : 3, and PM6:PCBM 2 : 8 nanoparticles in aqueous suspensions. (a) Transient absorption spectra of PM6 NPs at different time delays following excitation at 550 nm (fluence: 7.5μJ/cm2). (b) Comparison of transient absorption decay dynamics for neat PM6, PM6:PCBM 2 : 8, and PM6:Y6 7 : 3 NPs excited at 550 nm and probed at 1150 nm, assigned to PM6 exciton decay, with the long-lived residual signal assigned to PM6 polaron decay in the heterojunction NPs. (c, d) Transient absorption spectra of PM6:PCBM 2 : 8 NPs (c) and PM6:Y6 7 : 3 NPs (d) at different time delays, also excited at 550 nm (fluence: 7.5μJ/cm2). The disconnect in the transient absorption spectra axis corresponds to the pump laser scattering (550 nm) and the switch from visible to NIR detector (800–850 nm). (e) Schematic representation of exciton decay and electron/energy transfer processes in these NPs.
References
[1] X C Wang, K Maeda, A Thomas et al. A metal-free polymeric photocatalyst for hydrogen production from water under visible light. Nat Mater, 8, 76(2009).
[2] R G Li, F X Zhang, D E Wang et al. Spatial separation of photogenerated electrons and holes among {010} and {110} crystal facets of BiVO4. Nat Commun, 4, 1432(2013).
[3] T Takata, J Z Jiang, Y Sakata et al. Photocatalytic water splitting with a quantum efficiency of almost unity. Nature, 581, 411(2020).
[4] H Nishiyama, T Yamada, M Nakabayashi et al. Photocatalytic solar hydrogen production from water on a 100-m2 scale. Nature, 598, 304(2021).
[5] X Y Wang, L J Chen, S Y Chong et al. Sulfone-containing covalent organic frameworks for photocatalytic hydrogen evolution from water. Nat Chem, 10, 1180(2018).
[6] Y Bai, L Wilbraham, H Gao et al. Photocatalytic polymers of intrinsic microporosity for hydrogen production from water. J Mater Chem A, 9, 19958(2021).
[7] J Kosco, F Moruzzi, B Willner et al. Photocatalysts based on organic semiconductors with tunable energy levels for solar fuel applications. Adv Energy Mater, 10, 2001935(2020).
[8] C H Dai, B Liu. Conjugated polymers for visible-light-driven photocatalysis. Energy Environ Sci, 13, 24(2020).
[9] G Itskos, G Heliotis, P G Lagoudakis et al. Efficient dipole-dipole coupling of Mott-Wannier and Frenkel excitons in (Ga, In)N quantum well/polyfluorene semiconductor heterostructures. Phys Rev B, 76, 035344(2007).
[10] C Z Li, J L Liu, H Li et al. Covalent organic frameworks with high quantum efficiency in sacrificial photocatalytic hydrogen evolution. Nat Commun, 13, 2357(2022).
[11] J Kosco, S Gonzalez-Carrero, C T Howells et al. Generation of long-lived charges in organic semiconductor heterojunction nanoparticles for efficient photocatalytic hydrogen evolution. Nat Energy, 7, 340(2022).
[12] M M Ma, Y B Huang, J Liu et al. Engineering the photoelectrochemical behaviors of ZnO for efficient solar water splitting. J Semicond, 41, 091702(2020).
[13] Y F Zhong, M Causa', G J Moore et al. Sub-picosecond charge-transfer at near-zero driving force in polymer: Non-fullerene acceptor blends and bilayers. Nat Commun, 11, 833(2020).
[14] J Wang, D Liu, Y F Zhu et al. Supramolecular packing dominant photocatalytic oxidation and anticancer performance of PDI. Appl Catal B, 231, 251(2018).
[15] N Zhang, L Wang, H M Wang et al. Self-assembled one-dimensional porphyrin nanostructures with enhanced photocatalytic hydrogen generation. Nano Lett, 18, 560(2018).
[16] C J Laconsay, K Y Tsui, D J Tantillo. Tipping the balance: Theoretical interrogation of divergent extended heterolytic fragmentations. Chem Sci, 11, 2231(2020).
[17] P Peng, X X Yan, K Zhang et al. Electrochemical C−C bond cleavage of cyclopropanes towards the synthesis of 1, 3-difunctionalized molecules. Nat Commun, 12, 3075(2021).
[18] M Y Wang, M Li, S Yang et al. Radical-mediated C-C cleavage of unstrained cycloketones and DFT study for unusual regioselectivity. Nat Commun, 11, 672(2020).
[19] P Liu, E Redekop, X Gao et al. Oligomerization of light olefins catalyzed by Brønsted-acidic metal-organic framework-808. J Am Chem Soc, 141, 11557(2019).