Abstract
An amplified and narrow-linewidth distributed feedback fiber laser in a master oscillator power amplifier (MOPA) configuration is presented. It consists of a distributed feedback fiber laser as the seed laser and an MOPA structure in an all-single-mode fiber system. The optimized narrow-linewidth fiber laser has an over 15% efficient slope. The packaged laser module shows excellent performance including a narrow linewidth of 2 kHz, low relative intensity noise below at the relaxation oscillation frequency, and low phase noise, which make it very suitable for interference and distributed sensing applications.A stable fiber laser source with narrow linewidth is the key component of a high-performance optical fiber interference sensing system and distributed sensing system[1]. A single-frequency narrow-linewidth fiber laser can be obtained in a ring cavity structure or a linear cavity structure. There are two types, such as the distributed Bragg reflector fiber laser (DBR-FL)[2] and the distributed feedback fiber laser (DFB-FL)[3] in the linear cavity structure. For the DBR-FL and DFB-FL, the very short cavity on an Er-doped fiber (EDF) is needed to meet the mode selection condition for single-frequency operation. Hence, they usually have a relatively low output power, below 1 mW, which makes it not practical as the laser source. In this paper, a narrow-linewidth DFB-FL with a master oscillator power amplifier (MOPA) structure having excellent characteristics and tens of milliwatts of power is presented.
The amplified DFB-FL consists of an Er-doped DFB-FL as the seed laser and a MOPA structure in an all-single-mode fiber system. The gain and oscillation cavity of the DFB-FL is a phase-shifted fiber grating fabricated by UV laser scanning and phase mask dithering on the EDF. It has excellent characteristics, including robust single-frequency operation, narrow linewidth, and low relative-intensity noise. The residual pump from the DFB-FL is utilized for subsequent optical amplification. The seed laser gets a high gain of 20 dB under a total of 300 mW 980 nm pump. The amplified DFB-FL with a 57 dB signal-to-noise ratio (SNR) (over 70 dB SNR after filter) has a linewidth of 2 kHz, a low-relative-intensity noise below at the relaxation oscillation frequency, and a low phase noise. These excellent characteristics make it attractive in distributed sensing and interference sensing applications.
A DFB-FL, has been comprehensively studied in theory and experiments for its important use in sensor applications, either as sensing elements or as sources for interrogation since the first report of this kind of fiber laser in the early 1990s[4]. The significant advantages include robust single-mode operation, ultra-narrow linewidth, and appropriateness for dense wavelength division multiplexing of sensor arrays[5].
Sign up for Chinese Optics Letters TOC. Get the latest issue of Chinese Optics Letters delivered right to you!Sign up now
The DFB-FL consists of a continuous Bragg grating with a phase shift written into a piece of EDF that is pumped by a 980 nm LD series pump. By introducing the phase-shift to the center of the grating, the grating resonance (equivalent to the resonance wavelength of Fabry–Perot cavity) is moved to the center of the grating reflection band, and the resulting DFB-FL can operate very robustly in a single longitudinal mode. The schematic diagram for a DFB-FL is shown in Fig. 1.
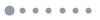
Figure 1.Structure of DFB-FL.
The phase-shifted grating for this DFB-FL is 40 mm in length and has a phase shift at the 0.4*Lg position, where Lg denotes the total length of the phase-shifted grating. The asymmetrical position for the phase shift leads to nearly unidirectional laser output and the DFB-FL has a slope efficiency of 0.15% with a 980 nm LD pump. The grating in the EDF with a precise phase shift is fabricated by an UV laser scanning and phase mask dithering technique, which has been described in previous publications[6,7].
In the normal DFB-FL structure, the EDF loading phase-shifted grating is short and only absorbs a small part of the incident pump laser. The laser output power is relatively low and not suitable for practical application in sensing systems. Hence, the residual pump laser is utilized for the subsequent amplification in the MOPA structure. The total structure is shown in Fig. 2. A 3 m-long EDF (Er-doped concentration: 4000 ppm) with absorption of 7 dB/m at 980 nm is used and optimized for power in the experiments. The laser output curve vs. pump is shown in Fig. 3(a). In this structure, two wavelength division multiplexers (WDMs) (WDM2 and WDM3) are used after the phase-shifted grating to separate the residual pump laser and the signal laser, while an isolator (ISO1) is applied after WDM2 to isolate the backward amplified spontaneous emission (ASE) from the pumped EDF so as to reduce the spectral noise. A net amplification of 20 dB can be achieved in this structure. To get a stable laser source, the DFB-FL is electrically temperature controlled and a compact package with sound isolation and vibration damping is applied. In the experiment, when different pump power from 10 to 300 mW is launched, no change about the linewidth is found. The final laser output is systematically tested. The characteristics of the laser are listed in Table 1 and shown in Figs. 3 to 5. For comparison the linewidth and phase noise of two commercial narrow-linewidth fiber lasers are tested. In Fig. 4, NKT C15[8] denotes a type of narrow-linewidth fiber laser products by NKT Photonics, Inc. and NP Rock[9] denotes one from NP Photonics, Inc.
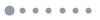
Figure 2.Schematic diagram for the DFB-FL with MOPA structure.
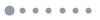
Figure 3.(a) Laser output curve and (b) laser spectra.
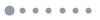
Figure 4.Characteristic comparisons of the DFB-FL with MOPA structure and other narrow-linewidth fiber lasers: (a) linewidth, self-homodyne method with 30 km OPD, (b) phase noise, 30 m OPD, unit: pm/Hz.
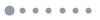
Figure 5.Narrow-linewidth DFB module.
Pump power | Laser output power (including ASE) | Relative intensity noise | Linewidth | Net power amplification (excluding ASE) |
---|
300 mW | 60 mW | −107 dB/Hz | 2 kHz | 20 dB |
Table 1. Characteristics of the DFB-FL with MOPA Structure
Based on the DFB-FL and MOPA structure, a narrow-linewidth fiber laser with low phase noise is obtained. Up to 60 mW of laser output is achieved after optimization. A compact laser module providing a stable laser output is presented. By comparison, it is proved that the narrow-linewidth DFB-FL has a narrower linewidth and lower phase noise than the NKT C15 and NP Rock products. These excellent characteristics make it very suitable and show great potential in interference and distributed sensing applications.
References
[1] G. A. Cranch, G. M. H. Flockhart, C. K. Kirkendall. Proc. SPIE, 6619, 66192C(2007).
[2] A. C. Wong, D. Chen, H. Wang, W. H. Chung, H. Y. Tam, C. Lu, B. Guan. Meas. Sci. Technol., 22, 045202(2011).
[3] M. Sejka, P. Varming, J. Hubner, M. Kristensen. Electron. Lett., 31, 1445(1995).
[4] J. T. Kringlebotn, J. L. Archambault, L. Reekie, D. N. Payne. Opt. Lett., 19, 2101(1994).
[5] S. Foster, A. Tikhomirov, M. Englund. Proceedings of the Australian Conference on Optical Fibre Technology (ACOFT’06) ACOFT/AOS 2006, 40-42(2006).
[6] H. Qi, Z. Song, S. Li, C. Wang, G. Peng. Chin. Opt. Lett., 11, 041407(2013).
[7] H. Qi, Z. Song, J. Guo, C. Wang, G. Peng. Opt. Express, 21, 11309(2013).
[8] . Koheras Basik C15–Ultra low noise, single frequency fiber laser modules in the 1.5 μm range.
[9] . Rock module compact single-frequency fiber laser.