
- Photonics Research
- Vol. 10, Issue 9, 2133 (2022)
Abstract
1. INTRODUCTION
Quantum dot light-emitting diodes (QLEDs) have been regarded as one of the most promising alternatives for the next generation of display and lighting devices because of their high photoluminescence quantum yield (PL QY), size-controllable emission wavelength, and simple solution processability [1–9]. At present, the QLEDs based on CdSe and perovskite quantum dots (QDs) have greatly improved, and their performance can be comparable to the commercial organic light-emitting diodes (OLEDs) [10–14]. However, according to the “Restriction of Hazardous Substances Directive”, the inherent toxicity of Cd/Pb might inhibit their further development. Indium phosphide (InP) QDs have become the most promising benign substitute for heavy metal-free emitters due to their excellent luminescence properties [15–19]. Although some breakthroughs have been achieved in the electroluminescence (EL) of InP-based QLEDs through continuous efforts and attempts, their performance is still falling behind those of Cd- and Pb-based QLEDs [10,11,15,19]. Therefore, it is necessary to exploit highly efficient InP-based QLEDs with considerable stability for future display applications.
To improve the efficiency and stability of InP-based QLEDs, researchers focused on synthesizing high PL QY InP QDs and modifying the device structures [15,19]. However, it should be noted that almost all reports of InP-based QLEDs are using polystyrene sulfonate (PEDOT:PSS) as the hole injection layer (HIL) at present [17,20–22]. The water-based PEDOT:PSS dispersion has side effects on the stability of QLEDs due to its hygroscopicity and the acidity that corrodes the indium tin oxide (ITO) electrode, thus shortening the lifetime of QLEDs [23]. It is reported that p-type metal oxide (
The balance between electron and hole injection into the QD emission layer is significant to acquire high-performance QLEDs [18,28–30]. Due to the deep valence band (VB) of QDs, hole injection is generally more difficult than electron injection because of the higher injection barrier [3]. The use of bilayered HIL is a good way to reduce the energy barrier at each step. Scientists have conducted research in the field of OLEDs to improve their efficiency [12,13]. However, the multilayered HIL for QLEDs, especially the multilayered HIL around all inorganic materials, has rarely been studied. It is also reported that the electronic properties of
Sign up for Photonics Research TOC. Get the latest issue of Photonics Research delivered right to you!Sign up now
In the present work, we report bilayered HIL InP-based QLEDs prepared by solution processing of colloidal
2. EXPERIMENTAL DETAILS
A. Materials
B. Synthesis of
The colloidal
C. Synthesis of Mg-Doped ZnO NCs
40 mL of DMSO was combined with 8.5 mmol of
D. Fabrication of QLEDs
The QLEDs were fabricated based on the structure of the ITO/HIL layers (PEDOT:PSS,
The hole-only device with a structure of the ITO/HIL layers (PEDOT:PSS,
E. Characterization
The transmission electron microscope (TEM) images were obtained using a JEOL JEM 2100PLUS microscope working at 200 kV. The X-ray diffraction (XRD) was measured using a
3. RESULTS AND DISCUSSION
The bilayered HILs were prepared by solution processing of colloidal
Figure 1.Structure, optical bandgap, and energy level of
While the energy levels of the solution-processed
The surface morphology and optical transmittance of the HIL film prepared by solution processing are very important to the properties of QLEDs. Figures 2(a)–2(c) show AFM images of
Figure 2.(a)–(c) AFM images of the (a) ITO/NiO, (b)
To investigate effect of the bilayered HIL on the EL performance in the devices, the QLEDs were constructed with a typical structure of
Figure 3.(a) Schematic of the layers in the device structure; (b) energy level diagram of the devices; (c) normalized EL spectra of devices. The inset shows a photograph of a device. (d) Luminance–voltage–current density (
Figure 3(d) shows the current density–luminance–voltage (
Summary of EL Performances of Devices with Different Structures
Device Structure | FWHM (nm) | CE ( | EQE (%) | ||
---|---|---|---|---|---|
ITO/PEDOT:PSS/poly-TPD/QDs/ZnMgO/Al | 629 | 46 | 9.9 | 7.6 | 7519 |
629 | 46 | 10.6 | 8.1 | 9704 | |
629 | 46 | 15.4 | 11.2 | 29,445 |
To understand the change in carrier transport properties caused by the
Figure 4.(a)–(c) Charged
4. CONCLUSION
The potential barrier of hole injection is large due to the low VB energy level of QDs, and the resulting charge balance difference is a typical feature of QLEDs. To alleviate the imbalanced charge injection, we introduced the
References
[1] J. C. Loudon, N. D. Mathur, P. A. Midgley. Charge-ordered ferromagnetic phase in La0.5Ca0.5MnO3. Nature, 420, 797-800(2002).
[2] K.-S. Cho, E. K. Lee, W.-J. Joo, E. Jang, T.-H. Kim, S. J. Lee, S.-J. Kwon, J. Y. Han, B.-K. Kim, B. L. Choi, J. M. Kim. High-performance crosslinked colloidal quantum-dot light-emitting diodes. Nat. Photonics, 3, 341-345(2009).
[3] L. Qian, Y. Zheng, J. Xue, P. H. Holloway. Stable and efficient quantum-dot light-emitting diodes based on solution-processed multilayer structures. Nat. Photonics, 5, 543-548(2011).
[4] Y. Shirasaki, G. J. Supran, M. G. Bawendi, V. Bulović. Emergence of colloidal quantum-dot light-emitting technologies. Nat. Photonics, 7, 13-23(2012).
[5] X. Dai, Z. Zhang, Y. Jin, Y. Niu, H. Cao, X. Liang, L. Chen, J. Wang, X. Peng. Solution-processed, high-performance light-emitting diodes based on quantum dots. Nature, 515, 96-99(2014).
[6] H. Zhang, S. Chen, X. W. Sun. Efficient red/green/blue tandem quantum-dot light-emitting diodes with external quantum efficiency exceeding 21%. ACS Nano, 12, 697-704(2018).
[7] H. Shen, Q. Gao, Y. Zhang, Y. Lin, Q. Lin, Z. Li, L. Chen, Z. Zeng, X. Li, Y. Jia, S. Wang, Z. Du, L. S. Li, Z. Zhang. Visible quantum dot light-emitting diodes with simultaneous high brightness and efficiency. Nat. Photonics, 13, 192-197(2019).
[8] S. Cao, J. Zheng, J. Zhao, Z. Yang, C. Li, X. Guan, W. Yang, M. Shang, T. Wu. Enhancing the performance of quantum dot light-emitting diodes using room-temperature-processed Ga-doped ZnO nanoparticles as the electron transport layer. ACS Appl. Mater. Interfaces, 9, 15605-15614(2017).
[9] S. Yu, Y. Tang, Z. Li, K. Chen, X. Ding, B. Yu. Enhanced optical and thermal performance of white light-emitting diodes with horizontally layered quantum dots phosphor nanocomposites. Photon. Res., 6, 90-98(2018).
[10] D. Chen, D. Chen, X. Dai, Z. Zhang, J. Lin, Y. Deng, Y. Hao, C. Zhang, H. Zhu, F. Gao, Y. Jin. Shelf-stable quantum-dot light-emitting diodes with high operational performance. Adv. Mater., 32, 2006178(2020).
[11] D. Liu, S. Cao, S. Wang, H. Wang, W. Dai, B. Zou, J. Zhao, Y. Wang. Highly stable red quantum dot light-emitting diodes with long
[12] A. Yonish, R. Shikler. The influence of the internal interface energy barrier and the device dimensions on the transient electroluminescence lifetime of bi-layer OLEDs. J. Mater. Chem. C, 10, 7141-7146(2022).
[13] M. Kim, B. H. Kwon, C. W. Joo, M. S. Cho, H. Jang, Y. J. Kim, H. Cho, D. Y. Jeon, E. N. Cho, Y. S. Jung. Metal oxide charge transfer complex for effective energy band tailoring in multilayer optoelectronics. Nat. Commun., 13, 75(2022).
[14] N. Zhang, X. Qu, Q. Lyu, K. Wang, X. W. Sun. Highly efficient transparent quantum-dot light-emitting diodes based on inorganic double electron-transport layers. Photon. Res., 9, 1979-1983(2021).
[15] Y. H. Won, O. Cho, T. Kim, D. Y. Chung, T. Kim, H. Chung, H. Jang, J. Lee, D. Kim, E. Jang. Highly efficient and stable InP/ZnSe/ZnS quantum dot light-emitting diodes. Nature, 575, 634-638(2019).
[16] Y. Li, X. Hou, X. Dai, Z. Yao, L. Lv, Y. Jin, X. Peng. Stoichiometry-controlled InP-based quantum dots: synthesis, photoluminescence, and electroluminescence. J. Am. Chem. Soc., 141, 6448-6452(2019).
[17] H. Zhang, N. Hu, Z. Zeng, Q. Lin, F. Zhang, A. Tang, Y. Jia, L. S. Li, H. Shen, F. Teng, Z. Du. High-efficiency green InP quantum dot-based electroluminescent device comprising thick-shell quantum dots. Adv. Opt. Mater., 7, 1801602(2019).
[18] Q. Su, H. Zhang, S. Chen. Identification of excess charge carriers in InP-based quantum-dot light-emitting diodes. Appl. Phys. Lett., 117, 053502(2020).
[19] H. Li, W. Zhang, Y. Bian, T. K. Ahn, H. Shen, B. Ji. ZnF2-assisted synthesis of highly luminescent InP/ZnSe/ZnS quantum dots for efficient and stable electroluminescence. Nano Lett., 22, 4067-4073(2022).
[20] L. Wang, J. Lin, X. Liu, S. Cao, Y. Wang, J. Zhao, B. Zou. Mg-Doped ZnO nanoparticle films as the interlayer between the ZnO electron transport layer and InP quantum dot layer for light-emitting diodes. J. Phys. Chem. C, 124, 8758-8765(2020).
[21] P. Yu, Y. Shan, S. Cao, Y. Hu, Q. Li, R. Zeng, B. Zou, Y. Wang, J. Zhao. Inorganic solid phosphorus precursor of sodium phosphaethynolate for synthesis of highly luminescent InP-based quantum dots. ACS Energy Lett., 6, 2697-2703(2021).
[22] M. G. Han, Y. Lee, H.-I. Kwon, H. Lee, T. Kim, Y.-H. Won, E. Jang. InP-based quantum dot light-emitting diode with a blended emissive layer. ACS Energy Lett., 6, 1577-1585(2021).
[23] F. So, D. Kondakov. Degradation mechanisms in small-molecule and polymer organic light-emitting diodes. Adv. Mater., 22, 3762-3777(2010).
[24] F. Cao, H. Wang, P. Shen, X. Li, Y. Zheng, Y. Shang, J. Zhang, Z. Ning, X. Yang. High-efficiency and stable quantum dot light-emitting diodes enabled by a solution-processed metal-doped nickel oxide hole injection interfacial layer. Adv. Funct. Mater., 27, 1704278(2017).
[25] F. Wang, Z. Wang, X. Zhu, Y. Bai, Y. Yang, S. Hu, Y. Liu, B. You, J. Wang, Y. Li, Z. Tan. Highly efficient and super stable full-color quantum dots light-emitting diodes with solution-processed all-inorganic charge transport layers. Small, 17, 2007363(2021).
[26] S. Rhee, D. Hahm, H. J. Seok, J. H. Chang, D. Jung, M. Park, E. Hwang, D. C. Lee, Y. S. Park, H. K. Kim, W. K. Bae. Steering interface dipoles for bright and efficient all-inorganic quantum dot based light-emitting diodes. ACS Nano, 15, 20332-20340(2021).
[27] F. Cao, Q. Wu, Y. Sui, S. Wang, Y. Dou, W. Hua, L. Kong, L. Wang, J. Zhang, T. Jiang, X. Yang. All-inorganic quantum dot light-emitting diodes with suppressed luminance quenching enabled by chloride passivated tungsten phosphate hole transport layers. Small, 17, 2100030(2021).
[28] Y. Deng, X. Lin, W. Fang, D. Di, L. Wang, R. H. Friend, X. Peng, Y. Jin. Deciphering exciton-generation processes in quantum-dot electroluminescence. Nat. Commun., 11, 2309(2020).
[29] Q. Wu, X. Gong, D. Zhao, Y. B. Zhao, F. Cao, H. Wang, S. Wang, J. Zhang, R. Quintero-Bermudez, E. H. Sargent, X. Yang. Efficient tandem quantum-dot LEDs enabled by an inorganic semiconductor-metal-dielectric interconnecting layer stack. Adv. Mater., 34, 2108150(2022).
[30] Q. Wu, F. Cao, S. Wang, Y. Wang, Z. Sun, J. Feng, Y. Liu, L. Wang, Q. Cao, Y. Li, B. Wei, W. Y. Wong, X. Yang. Quasi-shell-growth strategy achieves stable and efficient green InP quantum dot light-emitting diodes. Adv. Sci., 9, 2200959(2022).
[31] W. Ji, H. Shen, H. Zhang, Z. Kang, H. Zhang. Over 800% efficiency enhancement of all-inorganic quantum-dot light emitting diodes with an ultrathin alumina passivating layer. Nanoscale, 10, 11103-11109(2018).
[32] C.-Y. Han, S.-H. Lee, S.-W. Song, S.-Y. Yoon, J.-H. Jo, D.-Y. Jo, H.-M. Kim, B.-J. Lee, H.-S. Lee, H. Yang. More than 9% efficient ZnSeTe quantum dot-based blue electroluminescent devices. ACS Energy Lett., 5, 1568-1576(2020).
[33] Y. Liang, L. Jiang, F. S. Y. Yeung, P. Xu, S. Chen, H. S. Kwok, G. Li. All-inorganic quantum-dot light-emitting diodes with reduced exciton quenching by a MgO decorated inorganic hole transport layer. ACS Appl. Mater. Interfaces, 11, 11119-11124(2019).
[34] H. Du, L. Ma, X. Wang, Y. Li, M. Xu, X. Liang, D. Chen, Y. Jin. Synthesis of Cu-modified nickel oxide nanocrystals and their applications as hole-injection layers for quantum-dot light-emitting diodes. Chem. Eur. J., 27, 11298-11302(2021).
[35] R. He, S. Nie, X. Huang, Y. Wu, R. Chen, J. Yin, B. Wu, J. Li, N. Zheng. Scalable preparation of high-performance ZnO-SnO2 cascaded electron transport layer for efficient perovskite solar modules. Sol. RRL, 6, 2100639(2021).
[36] S.-H. Song, J.-I. Yoo, H.-B. Kim, Y.-S. Kim, S. S. Kim, J.-K. Song. Hole injection improvement in quantum-dot light-emitting diodes using bi-layered hole injection layer of PEDOT:PSS and
[37] R. Wang, T. Wang, Z. Kang, H. Zhang, R. Yu, W. Ji. Efficient flexible quantum-dot light-emitting diodes with unipolar charge injection. Opt. Express, 30, 15747-15756(2022).
[38] X. Xiao, T. Ye, J. Sun, X. Qu, Z. Ren, D. Wu, S. Ding, X. W. Sun, W. C. H. Choy, K. Wang. Capacitance-voltage characteristics of perovskite light-emitting diodes: modelling and implementing on the analysis of carrier behaviors. Appl. Phys. Lett., 120, 243501(2022).
[39] Y. Fang, P. Bai, J. Li, B. Xiao, Y. Wang, Y. Wang. Highly efficient red quantum dot light-emitting diodes by balancing charge injection and transport. ACS Appl. Mater. Interfaces, 14, 21263-21269(2022).
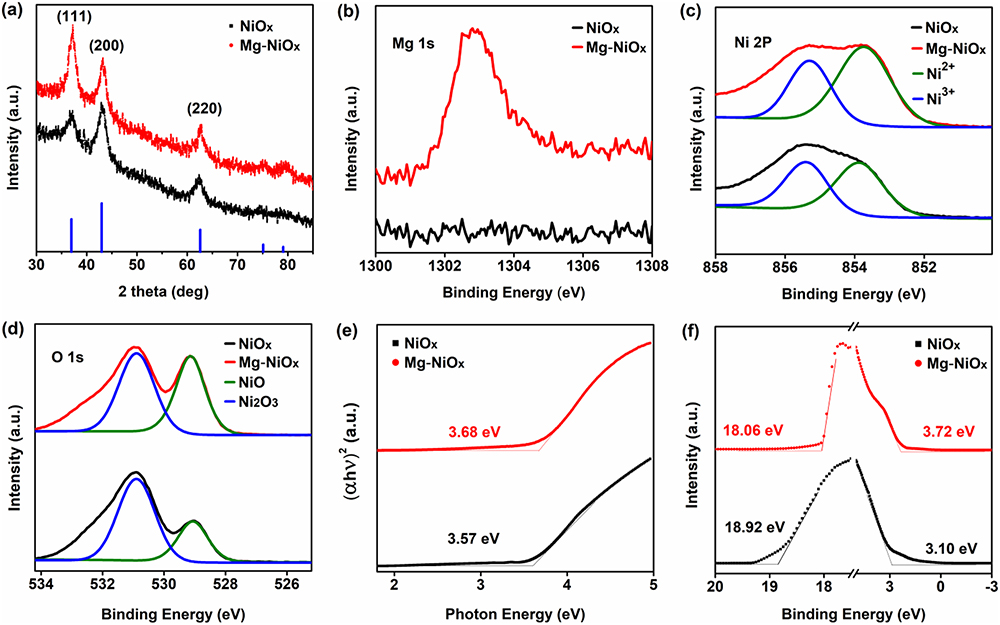
Set citation alerts for the article
Please enter your email address