
- Advanced Photonics
- Vol. 2, Issue 5, 050502 (2020)
Abstract
Guoqing Chang spoke to Shining Zhu of Nanjing University, about his insights into laser science, applications, and future prospects.
Figure 1.Shining Zhu
When did you first hear about laser?
As one of the greatest inventions of the 20th century, the laser has been applied to many fields, such as laser machining and processing, gravitational wave detection, precision metrology, laser spectroscopy and microscopy, 5G\6G technology, microwave photonics, just to name a few. I cannot remember the exact time I heard about lasers, but I have definitely read articles about them in popular magazines ever since I was young. It was around that time when I learned about the Chinese scientists who had developed lasers shortly after Theodore Maiman. In college, I majored in condensed matter physics and took courses on optics, from which I learned more about lasers and became more interested in the topic. The real exposure to the laser came when I needed to use a laser to study phase transition in some crystal materials during my pursuit of a master’s degree at Nanjing University. After earning the master’s degree, I joined a research group led by Professor Naiben Ming to pursue a PhD degree. My PhD research focused on the development of new optical structures in crystals to manipulate and control laser light.
Sign up for Advanced Photonics TOC. Get the latest issue of Advanced Photonics delivered right to you!Sign up now
In your research, laser is an experimental tool for material characterization, right?
This was the case at the beginning of my career. We started to take interest in the development of lasers as we discovered that nonlinear crystals with proper optical superlattice were suitable to achieve nonlinear frequency conversion. Such a capability allowed us to convert a laser’s original wavelength to new wavelengths, even leading to a laser system that output three colors (red, green, and blue) simultaneously. In this scenario, quasi-white light was achieved. Later, we showed that the optical superlattice has unique advantages in the mid-infrared (IR) wavelength range. Mid-IR lasers hold promise for many important applications (e.g., biomedicine, environmental monitoring, and national security), but mid-IR laser crystals are rare. Therefore, wavelength conversion becomes necessary to obtain mid-IR laser light from a source laser operating at the near-IR wavelength range. We developed a high-power, widely tunable mid-infrared laser system using a specially designed optical superlattice to perform wavelength conversion from a pump laser of
Your research on the Fibonacci optical superlattice connects the well-known golden ratio in mathematics and the quasi-phase-matching in nonlinear optics. The results are really elegant. Can you give us more details?
Before 1960, due to the lack of strong light sources, nonlinear optics mainly remained in sporadic theoretical research. After the emergence of the laser in 1960, nonlinear optics developed rapidly in both theory and experiment. In 1962, Bloembergen (Nobel Prize laureate in Physics in 1981) and his team theoretically proposed the concept of quasi-phase-matching (QPM) in nonlinear optics; that is, in order to enhance nonlinear optical processes, phase matching can be achieved by periodically changing the sign of the nonlinear coefficient of an optical crystal. In general, the modulation period in nonlinear crystals corresponding to the realization of OPM in the wavelength range from visible to IR was usually on the order of a few to a few tens of microns, which was extremely hard to implement.
The experimental verification of QPM had been hindered due to the difficulty of material fabrication, until the late 1970s when the research team in Nanjing University grew the first
Throughout the middle 1980s, because a variety of novel optical effects were discovered from the crystals with the periodic domains, the concept of optical superlattice was formally proposed as a new category of micro-structured materials. In 1982, Dan Shechtman (Nobel Prize laureate in Chemistry in 2011) discovered quasicrystal structure in aluminium-manganese alloy. The discovery was reported in Physical Review Letters in late 1984. Ming and his group began to consider whether the nonlinear crystals with quasi-periodic domain structure (i.e., the quasi-periodic optical superlattice) can be used to achieve QPM frequency conversion. They theoretically predicted that in a quasi-periodic superlattice two or more QPM optical parametric processes can be realized simultaneously. This is different from a periodic superlattice in which only a single QPM condition may be satisfied. Thus, they proposed the concept of the multiple quasi–phase-matching (MQPM) accordingly. This theoretic result was published in Physical Review B in 1990 — the year when I joined Ming’s group as a PhD student.
As my thesis advisor, Ming asked me to experimentally fabricate such quasi-periodic structures that cannot be obtained using the previous crystal growth method for making periodic superlattice. After my efforts, I made progress in pattern poling LN and LT (
How did the work move to generate higher-order nonlinear processes in a crystal?
The previous work was to achieve a quasi-periodic superlattice, which can obtain high-efficiency SHG at multiple wavelengths. We wondered if it was possible to use one crystal to simultaneously generate SHG and third-harmonic generation (THG), or even other higher-order nonlinear processes. According to MQPM principle, we designed and prepared such a quasi-periodic superlattice crystal in which both the QPM conditions required for SHG and sum-frequency generation (SFG) can be met, thus allowing these two nonlinear processes to occur simultaneously. The cascade of SHG and SFG can effectively produce THG and this cascaded second-order nonlinear process corresponds to much higher conversion efficiency compared to THG from pure third-order nonlinearity. We submitted the manuscript to Science and surprisingly it was quickly accepted.
Communication between the Chinese and American communities became active after the 1980s. You visited Pennsylvania State University in the 1990s, right?
I was a visitor at the Material Research Lab (MRL) of Pennsylvania State University from 1996 to 1998. Their research on ferroelectric materials was second to none in the world, focusing on the piezoelectric properties of crystals and ceramics for sonar applications. In contrast, our superlattice research studied the domain structure in ferroelectric crystals targeting the applications in nonlinear optics. We learned from each other and realized that there are two types of domain structures—average domain structure and geometric domain structure—in ferroelectric and piezoelectric crystals. The material properties can be greatly improved, enhanced, and even designed by engineering the domain configurations in these crystals.
Through your narration, it seems that the imperfections in the crystal growing process resulted in unexpected periodic striation, which in turn led to the subsequent research in superlattice and QPM. Would you like to comment?
Many scientific discoveries originated from unexpected experimental phenomena, including Shechtman’s discovery of quasicrystals while studying aluminum-manganese alloys. This happened several times in my research group. For example, a graduate student did not find the expected laser light at the output end of a nonlinear crystal with specially designed superlattice structure; instead, he observed that some laser light appeared from the side surface at a special angle. After careful study, we found that this unexpected phenomenon corresponds to nonlinear Cherenkov radiation, which in fact represented the first observation of Cherenkov radiation in a nonlinear optical superlattice. The results were later published in the Physical Review Letters and the article was featured as the journal cover.
In another experiment, a student found that in addition to the round spot in the middle of the laser beam at the crystal output, rings of light appeared unexpectedly. After repeated experiments, we found that these rings came from elastic scattering caused by defects in the crystal. The superlattice structure of the crystal enhanced the scattering during the nonlinear optical process. It was extremely difficult to directly measure the elastic scattering inside a crystal. Our research provided a new method to characterize its spatial distribution in the crystal by measuring the angle, diameter, and intensity of these light rings.
It is quite common that new materials and novel structural designs are often accompanied by new discoveries and may even produce new physical concepts. This kind of unexpected discovery makes us feel that research, though sometimes exhausting, is a lot of fun.
What research fields are you currently focusing on?
Quantum information processing must be integrated on a chip to be practical, and therefore we are developing various technologies to enable large-scale integrated quantum photonics. We are the first to develop quantum chips based on superlattices. With the development of 5G\6G communications, we are also working hard to apply integration technology to microwave photonics, laser frequency combs, quantum simulations, etc. In addition, we utilize metasurfaces and artificial intelligence to develop new imaging technologies. For example, we successfully developed the first broadband achromatic metalens and the first
In the future, we will continue to use microstructures to engineer the optical properties of materials and fabricate functional devices to control the amplitude, phase, polarization, and angular momentum of a laser beam. I firmly believe that microstructure science holds promise for many prospective applications.
References
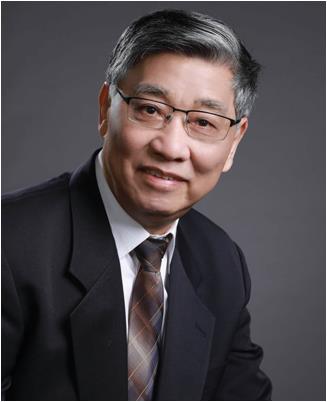
Set citation alerts for the article
Please enter your email address