
- Advanced Photonics
- Vol. 2, Issue 5, 050501 (2020)
Abstract
The Year 2020 represents 60 years since the first successful operation of the laser. This anniversary provides an ideal occasion to reflect on the myriad ways that lasers have revolutionized society, and to consider the many new areas of research that continue to drive photonics in unexpected directions. Yet at the same time as we consider these exciting future perspectives, it is also interesting to see how the development of the laser traces a path that intertwines basic and applied science, and intersects with the recognition of many of the pioneers of optics through the Nobel Prize. Of course, an exhaustive history of such a rich topic cannot be given in a short Perspective, but it is perhaps possible to describe some of the key highlights.
A good point to begin any historical discussion of the laser is the second half of 19th century, and the study of the emission properties of hot objects and the measurements of the characteristic spectrum of black body radiation. In fact, it is not widely appreciated that these studies were not initially motivated by questions of fundamental scientific curiosity, but were rather stimulated by a very practical and economic problem.
Although the initial measurements of blackbody radiation may have had a strong industrial link, the scientific environment of the time was clearly focused on understanding the deep and fundamental questions concerning the nature of light–matter interactions. Indeed, the Nobel Prize was first awarded only in 1901, and the importance of studying the nature of light was quickly recognized with prizes to Lorentz and Zeeman (1902), Wien (1911), and Planck himself (1918).
Sign up for Advanced Photonics TOC. Get the latest issue of Advanced Photonics delivered right to you!Sign up now
It was in 1917 when Einstein made a key contribution to the laser through his prediction of the process of Stimulated Emission.
Building on these ideas, researchers extended both theory and experiment of light–matter interactions during the following decades, leading to the development of the concepts such as pumping and resonators, and ultimately the first demonstration of the maser in 1953 by Charles Townes and his PhD student Jim Gordon. With Arthur Schawlow, in 1958 Townes wrote a theoretical paper extending the maser concept into the visible spectrum, although they had yet to build an experimental prototype.
Figure 1.(Left to right) James P. Gordon, Nikolai Basov, Herbert Zeiger, Alexander Prokhorov and Charles Hard Townes at the First Quantum Electronics Conference, Shawanga Lodge, September 14–16, 1959. Photo courtesy of The Regents of the University of California, Lawrence Berkeley National Laboratory.
The pioneering work on both masers and lasers was recognized in 1964 with the award of the Nobel Prize to Charles Townes, Nicolay Basov, and Aleksandr Prokhorov “for fundamental work in the field of quantum electronics, which has led to the construction of oscillators and amplifiers based on the maser-laser principle.” In fact, although many laser histories tend to focus mainly on the work performed in the USA, Basov and Prokhorov at the Lebedev Institute in Moscow were simultaneously and independently covering the same ground with their own theory and experiments. Two years later in 1966, Albert Kastler received the Nobel Prize for his work on optical pumping techniques, and later Bloembergen and Schawlow shared the 1981 Nobel Prize for laser applications in spectroscopy.
Maiman, despite being the first to see laser emission, never won the Nobel Prize, and neither did Jim Gordon. Whilst it is natural to consider these omissions as major oversights by the Nobel Committee, the available Nobel Prize archives
There were of course many other eminent scientists involved in the early years of laser physics, and some excellent personal and historical accounts are available.
Figure 2.A selection of Nobel Prizes related to light, masers and lasers, and applications. The descriptions are highly abridged from the formal citation, and this is of course only a partial list. Many other Nobel Prizes have involved key areas of light science. In particular, some physics Nobel Prizes in astronomy and cosmology include laser instrumentation as central components e.g. gravitational wave detection and laser interferometery (Nobel 2017) and the observation of black holes building on laser guide star adaptive optics (Nobel 2020). A printable poster version is included as Supplementary Material.
Considering the history of the laser is an opportunity to think about many broader issues of science, and particularly the relationship between basic research and technology transfer. The laser is an ideal subject with which to explain the tremendous economic and societal benefits that can arise from basic curiosity-driven scientific research. With all the advances in photonics that continue to be made in many different areas, it is likely that laser-related science will continue to be recognized by Nobel Prizes in the future, and will continue to create revolutions in our lives.
References
[1] H. Kubbinga. A tribute to Max Planck. Europhys. News, 49, 27-33(2018).
[4] A. Einstein. Zur Quantentheorie der Strahlung. Phys. Z., 18, 121-128(1917).
[5] C. H. Townes. How the Laser Happened: Adventures of a Scientist(1999).
[6] . Physics Today, 12, 72(1959).
[7] T. H. Maiman. The Laser Inventor: Memoirs of Theodore H. Maiman(2018).
[8]
[9]
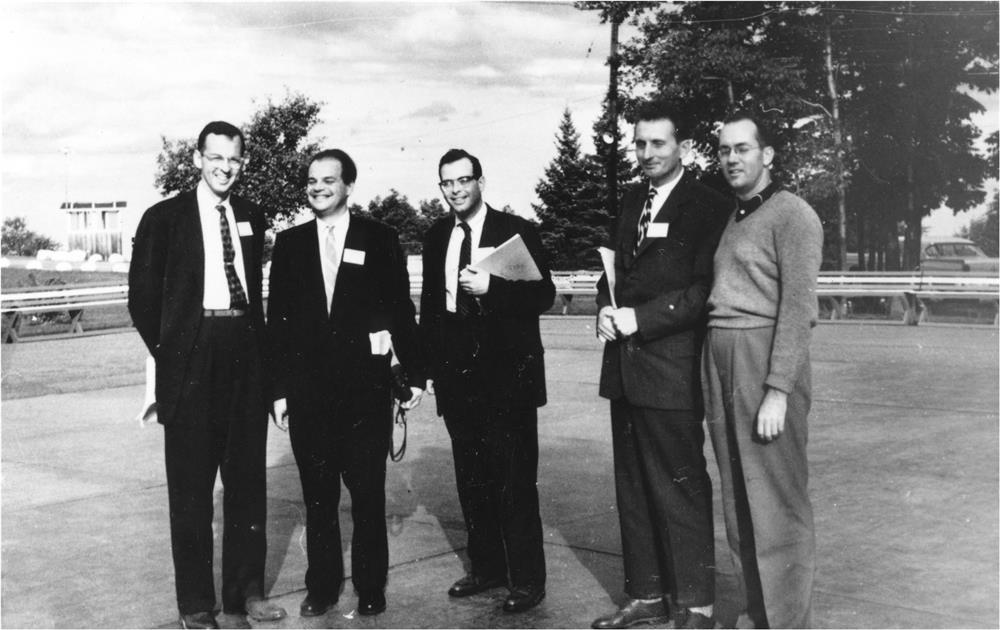
Set citation alerts for the article
Please enter your email address