Shenchao Jin, Han Bao, Junlei Duan, Xingda Lu, Mingfeng Wang, Kai-Feng Zhao, Heng Shen, Yanhong Xiao, "Adiabaticity in state preparation for spin squeezing of large atom ensembles," Photonics Res. 9, 2296 (2021)

Search by keywords or author
- Photonics Research
- Vol. 9, Issue 11, 2296 (2021)
![Experimental setup. (a) Schematics. The CSS nearly along the magnetic field in the x direction is created by optical pumping, with a pump laser tuned to the Rb D1 transition 5S1/2,F=2→5P1/2,F′=2 and a repump laser stabilized to the Rb D2 transition 5S1/2,F=1→5P3/2,F′=2, both with σ− circular polarization. A linearly polarized off-resonant D2 laser, propagating in the z direction, probes the quantum fluctuations of the spin. The Stokes component Sy of the probe laser is measured using a balanced polarimetry scheme and then detected by a spectrum analyzer or by a lock-in amplifier with demodulation frequency equal to the Larmor frequency ΩL. (b) Pulse sequence. The pump lasers are turned on to prepare the atoms into CSS, and are then turned off adiabatically (see text), followed by the probe pulse. The probe pulse detects the atomic Jz component after the pumping process. To evade the quantum back-action, the probe light is modulated stroboscopically (not shown in the figure) at twice the Larmor frequency [9,11].](/richHtml/prj/2021/9/11/11002296/img_001.jpg)
Fig. 1. Experimental setup. (a) Schematics. The CSS nearly along the magnetic field in the x direction is created by optical pumping, with a pump laser tuned to the Rb D1 transition 5 S 1 / 2 , F = 2 → 5 P 1 / 2 , F ′ = 2 and a repump laser stabilized to the Rb D2 transition 5 S 1 / 2 , F = 1 → 5 P 3 / 2 , F ′ = 2 , both with σ − circular polarization. A linearly polarized off-resonant D2 laser, propagating in the z direction, probes the quantum fluctuations of the spin. The Stokes component S y of the probe laser is measured using a balanced polarimetry scheme and then detected by a spectrum analyzer or by a lock-in amplifier with demodulation frequency equal to the Larmor frequency Ω L . (b) Pulse sequence. The pump lasers are turned on to prepare the atoms into CSS, and are then turned off adiabatically (see text), followed by the probe pulse. The probe pulse detects the atomic J z component after the pumping process. To evade the quantum back-action, the probe light is modulated stroboscopically (not shown in the figure) at twice the Larmor frequency [9,11].
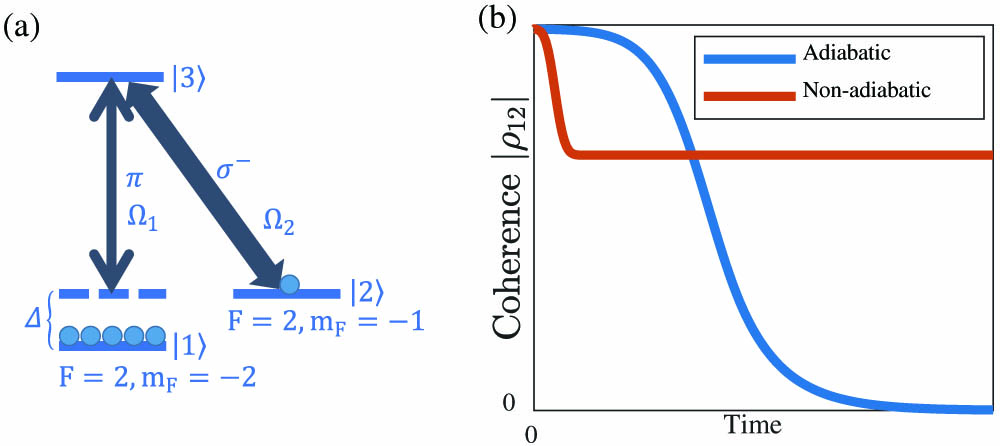
Fig. 2. (a) Simplified three-level Λ system. Δ is the two-photon detuning induced by Zeeman shift, Ω 1 and Ω 2 are the Rabi frequencies of the π and circular polarization components of the pump laser, respectively, where the circular polarization component is on one-photon resonance. Here, | 1 ⟩ = | F = 2 , m F = − 2 ⟩ , | 2 ⟩ = | F = 2 , m F = − 1 ⟩ . (b) Evolution of the ground-state coherence during the pump pulse’s falling edge. The blue line shows the case where the system is completely adiabatic. The red line corresponds to the nonadiabatic process for the quick falling edge.
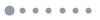
Fig. 3. (a) Calculated residual ground-state coherence | ρ 12 | versus the pump’s falling edge time for different Larmor frequencies. Coherence decreases as the falling edge time increases. Experiment data are denoted by the scattered dots, and the numerical calculation is shown by the dashed lines. The vertical axis for the numerical results is multiplied by a coefficient (same for all the four curves) to fit the data. The falling edge time of the experiment pulse is obtained by fitting the actual pump pulse’s falling edge by a Gaussian function. (b) Calculated residual ground-state coherence using the multilevel model with Doppler velocity integration. Scattered dots are the same experiment data as in (a).
![(a) Mean value of the measured signal for fast and slow falling edge of the pump lasers, respectively. An oscillation of 2 kHz appears for the sharp falling edge and nearly vanishes when the adiabatic pulse control is used. The oscillation is produced by atomic spin in F=1, with an oscillation frequency of 2 kHz (after demodulation by lock-in amplifier) originated from the Larmor frequency difference between the F=1 and F=2 Zeeman levels. (b) Theoretical result for the experimental curves in (a). (c) Variance of the measured signal for the sharp and slow falling edges of the pump lasers, at different times of the probe pulse. The variance is larger for the sharp falling edge because systematic instabilities turn large mean value into more extra classical noise. (d) Measured atom PN for the sharp and slow falling edges of the pump lasers as a function of duration of the probe pulse. The atomic noise here is extracted [9] from the measured total noise power integrated over the whole probe pulse. The adiabatic pump falling edge considerably decreases the detected noise level by 3 dB.](/Images/icon/loading.gif)
Fig. 4. (a) Mean value of the measured signal for fast and slow falling edge of the pump lasers, respectively. An oscillation of 2 kHz appears for the sharp falling edge and nearly vanishes when the adiabatic pulse control is used. The oscillation is produced by atomic spin in F = 1 , with an oscillation frequency of 2 kHz (after demodulation by lock-in amplifier) originated from the Larmor frequency difference between the F = 1 and F = 2 Zeeman levels. (b) Theoretical result for the experimental curves in (a). (c) Variance of the measured signal for the sharp and slow falling edges of the pump lasers, at different times of the probe pulse. The variance is larger for the sharp falling edge because systematic instabilities turn large mean value into more extra classical noise. (d) Measured atom PN for the sharp and slow falling edges of the pump lasers as a function of duration of the probe pulse. The atomic noise here is extracted [9] from the measured total noise power integrated over the whole probe pulse. The adiabatic pump falling edge considerably decreases the detected noise level by 3 dB.
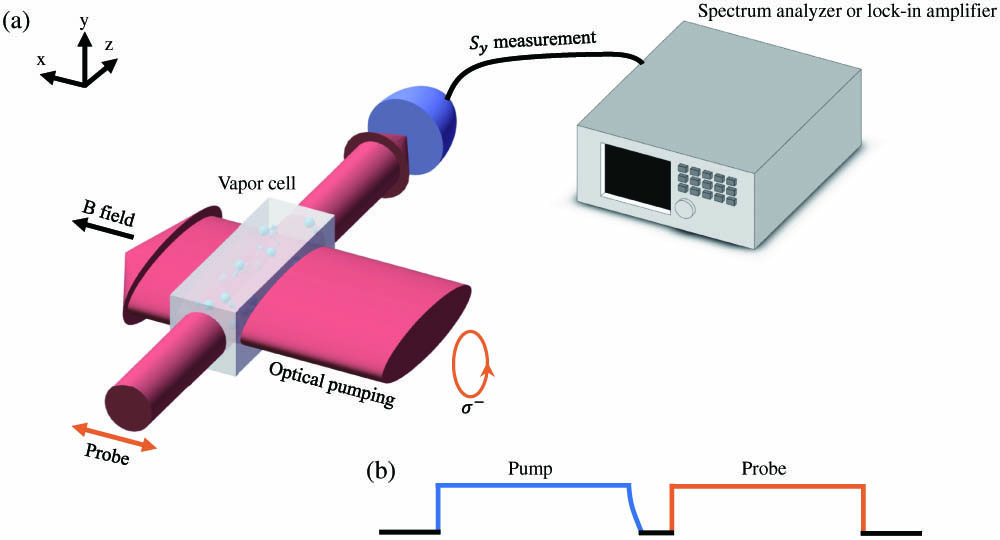
Set citation alerts for the article
Please enter your email address