Abstract
We report a simple solution-processed method for the fabrication of low-cost, flexible optical limiting materials based on graphene oxide (GO) impregnated polyvinyl alcohol (PVA) sheets. Such GO–PVA composite sheets display highly efficient broadband optical limiting activities for femtosecond laser pulses at 400, 800, and 1400 nm with very low limiting thresholds. Femtosecond pump–probe measurement results revealed that nonlinear absorption played an important role for the observed optical limiting activities. High flexibility and efficient optical limiting activities of these materials allow these composite sheets to be attached to nonplanar optical sensors in order to protect them from light-induced damage.1. INTRODUCTION
Nonlinear optical materials (optical limiters and saturable absorbers) play a significant role in the field of optics owing to their unprecedented ability to modulate lasers pulses. Over the past decades, significant research efforts have been devoted to the development of broadband optical limiting materials and related devices. Good optical limiting materials generally exhibit high transmittance at low input intensities but can attenuate intense laser pulses. Such materials are important for protecting human eyes and sensitive detectors from damage by high-intensity light beams. Effective optical limiting behaviors have been observed in various nanomaterials such as carbon nanotubes (CNTs) [1,2], fullerenes [3], quantum dots [4], and noble metal nanoparticles [5,6]. It was recently found that suspensions of two-dimensional material such as graphene [7,8], hydrogen exfoliated graphene [9], graphene oxide (GO) [10,11], and their composites with other materials [12–17] exhibited broadband optical limiting properties. In 2011, Lim et al. [15] reported a giant broadband nonlinear optical response for nanosecond laser pulses on functionalized GO nanostructure dispersion. Very recently, Liaros et al. also demonstrated broadband near infrared optical power limiting behaviors of few layered GO dispersed in different organic solvents under nanosecond laser pulses [18].
Nonlinear scattering and nonlinear absorption are two possible mechanisms responsible for optical limiting behaviors [3,6,10,19]. Most of the reported optical limiting studies were performed on nanomaterials dispersed in different solvents, in which solvent microbubbles-induced nonlinear scattering at higher intensities played an important role [1,3,5]. Thin film materials are more convenient to use but generally display strong saturable absorption [8,20]. For example, although suspensions of metal nanoparticles [5,6], graphene, and graphene–polymer composites [20–23] showed strong broadband optical limiting activities, their thin films generally exhibited strong saturable absorption behavior [8,20,24–27]. One major challenge is fabrication of stable and flexible thin film-based optical limiting devices for practical applications. Flexible and low-cost materials that exhibit high broadband nonlinear absorption are ideal choices. Previously, we have demonstrated that spin-coated GO thin films on glass or plastic substrates exhibited tunable broadband optical limiting response for femtosecond laser pulses, and the nonlinear optical response of GO could be tuned from nonlinear absorption to saturable absorption by partial reduction of GO [28]. However, these spin-coated GO films have a few drawbacks. First, GO tends to be reduced into reduced GO easily under normal light in the long run. Secondly, a significant amount of GO solution was wasted in the spin-coating process. Thirdly, the interaction of GO with glass or plastic surface is not very strong, which may result in the detachment of GO from the surface. Herein, we demonstrated a simple method for fabrication of flexible nonlinear optical films by impregnating GO into polyvinyl alcohol (PVA) polymer sheets using a solution-processing method. The prepared GO–PVA composite sheets have been characterized by ultraviolet–visible–near-infrared (UV–Vis–NIR) transmittance, Raman spectroscopy, and atomic force microscopy (AFM). Broadband optical limiting properties of as-prepared flexible GO–PVA sheets made of different GO/PVA weight ratios have been investigated by femtosecond Z-scan measurements at 400, 800, and 1400 nm. We have found that these flexible GO–PVA sheets exhibited excellent optical limiting properties. Femtosecond pump–probe results suggest that nonlinear absorption (excited state absorption or multiphoton absorption) of GO play an important role in the observed strong optical limiting activity.
2. PREPARATION OF GO-PVA SHEETS
GO was prepared by oxidative exfoliation of graphite using the Hummers and Offeman method [29]. The details of the synthesis procedures have been reported elsewhere [28]. After the exfoliation, GO sheets were purified several times in a water/ethanol mixture and finally dispersed in water with a concentration of 0.5 mg/mL. The AFM image of GO sheets showed that they were polydisperse, with size ranging from 300 nm to several micrometers [Fig. 1(a)]. GO was incorporated into flexible and transparent PVA sheets by a solution-processing method as shown in Fig. 1(b). Briefly, a 1 mL GO solution (0.5 mg/mL) was mixed with 10 mL of PVA solution (200 mg/mL PVA solution was prepared by stirring PVA powder in water for 12 h at 80°C, weight ratio of ) in a sample vial and then the mixture was poured into a Petri dish. The GO–PVA mixture in the Petri dish was dried by heating at 40°C overnight to obtain GO–PVA sheets by peeling off from the Petri dish. GO–PVA sheets of different GO/PVA weight ratios were prepared by using a similar procedure. The prepared GO–PVA sheets have thickness of using the same amount of PVA. The impregnation of GO into PVA sheets have several advantages over drop casting or spin-coating methods in terms of uniformity and reduced wastage of the materials [15,28]. The prepared GO–PVA sheets with different concentrations of GO have been characterized by UV–Vis–NIR transmittance and femtosecond nonlinear transmittance measurements.
Sign up for Photonics Research TOC. Get the latest issue of Photonics Research delivered right to you!Sign up now
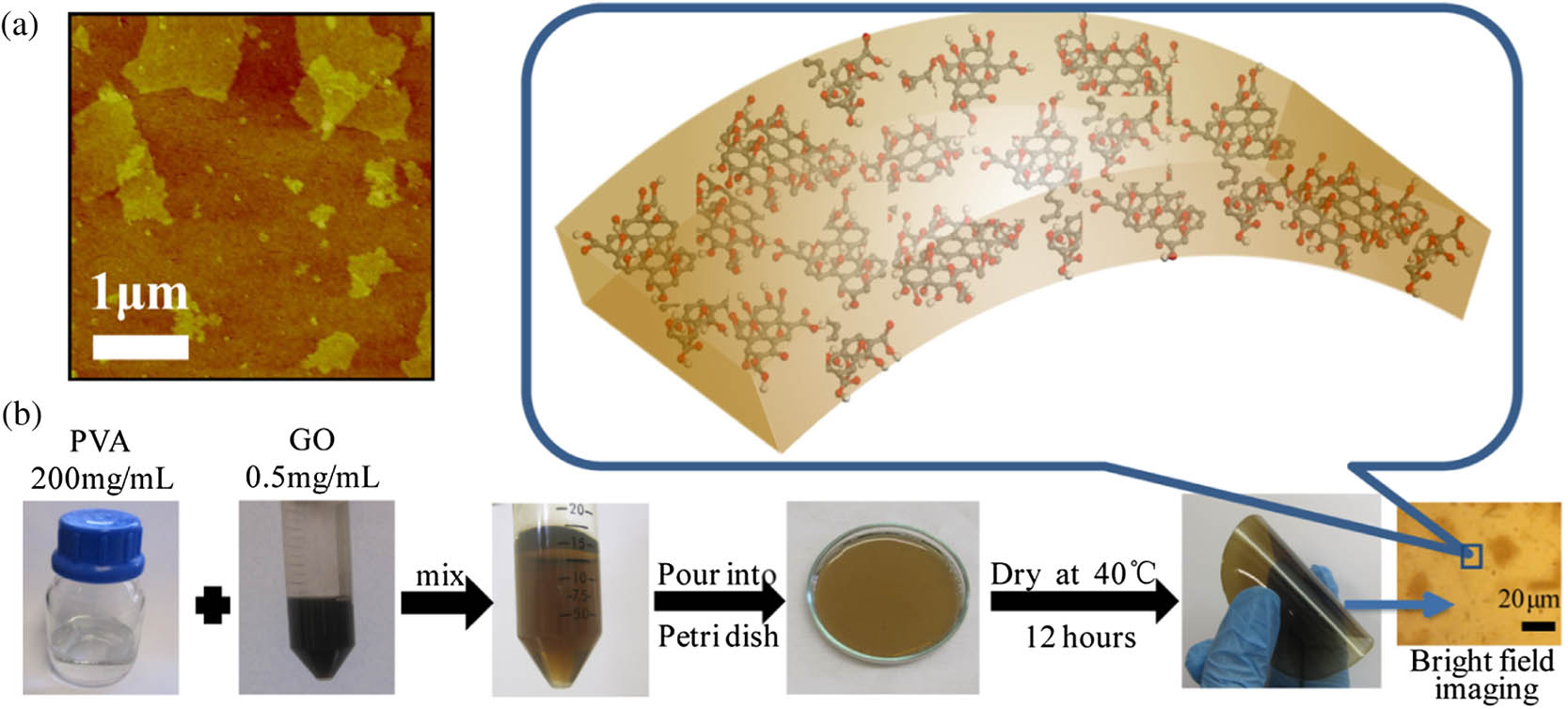
Figure 1.(a) AFM image of the as-prepared GO nanosheets. (b) Solution-processed preparation of flexible GO–PVA sheets by impregnating GO into PVA matrix and its bright field imaging. The inset is the schematic representation of GO–PVA sheets.
3. RESULTS AND DISCUSSION
Figure 2(a) shows the UV–Vis–NIR transmittance spectra of flexible GO–PVA sheets with different GO/PVA weight ratios. Similar to glass, a pure PVA sheet shows high transparency in the wavelength ranges from 400 to 900 nm. The GO–PVA sheets exhibit high transparency in the infrared region while the transmittance gradually decreases toward the UV region. As depicted in Fig. 2(a), the transmittance of the GO–PVA sheets decreases with increasing GO amount in PVA sheets. The optical properties of GO–PVA sheets are quite similar to GO films on glass substrates [15,28]. However, impregnation of GO inside the PVA polymer matrix in GO–PVA sheets helps to protect the GO from the outside environment considering the fact that GO can be easily reduced if exposed to the sunlight or reducing chemical vapors [28]. The Raman spectra of GO before and after the impregnation into PVA matrix are shown in Fig. 2(b). GO sheets exhibited two characteristic bands at and , corresponding to D (local defect band created by hydroxyl and epoxide groups on carbon plane) and G ( phonon mode of carbons) bands, respectively. The ratio of D/G increased from 0.73 to 0.92 after the incorporation of GO into PVA matrix, which might be attributed to the strong interactions of hydroxyl groups of GO with PVA.
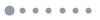
Figure 2.(a) UV–Vis–NIR transmittance spectra of GO–PVA sheets prepared with different GO/PVA weight ratios. (b) Raman spectra of GO before and after the impregnation into PVA sheet.
The nonlinear optical properties of prepared GO–PVA sheets have been investigated by using an open-aperture femtosecond Z-scan technique. The details of the experimental setup have been reported before [6,28]. Briefly, femtosecond laser pulses at 800 nm with pulse duration of 100 fs and repetition rate of 1 kHz were focused onto GO–PVA sheets, which were moved toward and away from the focus by using motorized translational stage to study the excitation fluence dependent transmission of GO–PVA sheets. Similar Z-scan measurements were also performed with femtosecond laser pulses at 400 nm, which were generated by focusing 800 nm laser pulses into a frequency doubling crystal. Figures 3(a) and 3(b) show the excitation fluence-dependent normalized transmittance of GO–PVA sheets at 400 and 800 nm, respectively (weight ratio of ). As depicted in Fig. 3(a), the normalized transmittance at 400 nm decreased as the sample moved toward the focus () at all excitation fluences. Such observation is quite interesting despite the fact that GO–PVA sheets have high linear absorption at 400 nm. Usually materials with high linear absorption exhibit saturable absorption at low excitation fluences due to ground-state bleaching. However, in the present case, we did not observe saturable absorption even at very low fluences at 400 nm excitation. This means that these GO–PVA sheets exhibit very high nonlinear absorption at 400 nm, which dominates the ground-state bleaching signal. However, in the case of 800 nm excitation [Fig. 3(b)], the normalized transmittance increased as the sample moved toward focus at low excitation fluence, which indicates saturable absorption occurred at low excitation fluence. As the excitation fluence increased, the Z-scan signals showed reverse saturable absorption. We have also performed Z-scan measurements on PVA sheets alone and they do not exhibit any optical limiting activity. The UV–Vis–NIR transmittance spectra and their optical limiting activities remained unchanged for at least half a year, indicating that PVA sheets protect GO from the environment to make these materials remarkably stable.
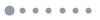
Figure 3.Open aperture Z-scan results of a GO–PVA sheet () at (a) 400 and (b) 800 nm under different excitation fluences. Optical limiting response of GO–PVA sheets of different GO/PVA weight ratios under femtosecond laser pulses at (c) 400 and (d) 800 nm, respectively.
Figures 3(c) and 3(d) show the excitation fluence-dependent normalized transmittance of GO–PVA sheets (different GO/PVA weight ratios) at 400 and 800 nm, respectively, which were extracted from the corresponding Z-scan results. The results show that the transmittance of GO–PVA sheets at both 400 and 800 nm decreased with the increasing input fluence, exhibiting broadband optical limiting activity. We have also performed the Z-scan measurements on pure PVA film alone and compared to the optical limiting properties of GO–PVA sheets. The results show that pure PVA sheets exhibit saturable absorption under 400 nm excitation due to ground-state bleaching of PVA. UV–Vis–NIR transmittance measurements [Fig. 2(a)] indicated that both GO and PVA have significant linear absorption at 400 nm. The fact that composite sheets exhibit optical limiting activity confirms the strong nonlinear absorption of GO at 400 nm. Under excitation at 800 nm, pure PVA alone does not show either saturable absorption or nonlinear absorption. There is thus no influence of PVA on the optical limiting properties of GO at 800 nm. The optical limiting thresholds (, the incident fluence at which the transmittance falls to 50% of the linear transmittance) and optical limiting onsets (, the input fluence where the transmittance starts to decrease) of GO–PVA sheets with different amount of GO are summarized in Table 1. The optical limiting thresholds of GO–PVA sheets at both 400 and 800 nm decreased with the increasing amount of GO in PVA matrix. The values of GO–PVA sheets are lower than the previously reported values for different materials. [6,30]
| | 800 nm | 400 nm | |
---|
Sample | Linear Transmittance | Optical Limiting Onset | Optical Limiting Threshold | Linear Transmittance | Optical Limiting Onset | Optical Limiting Threshold |
---|
Weight ratio (GO∶PVA) | T [%] | Fon [mJ·cm−2] | F50 [mJ·cm−2] | T [%] | Fon [mJ·cm−2] | F50 [mJ·cm−2] |
---|
0.00025∶1 | 97 | 4.0 | 73 | 63 | 3.7E−02 | 98 |
0.0005∶1 | 88 | 3.1 | 60 | 33 | 3.4E−02 | 27 |
0.001∶1 | 79 | 2.4 | 48 | 20 | 3.3E−02 | 7.7 |
0.0025∶1 | 40 | 2.2 | 40 | 2 | 2.7E−02 | 1.6 |
Table 1. Linear Transmittance (T), Optical Limiting Onsets () and Thresholds () of GO–PVA Sheets with Different Weight Ratios at 800 and 400 nm
We have also tested the optical limiting properties of a GO–PVA sheet () at 1400 nm by using the Z-scan measurements (Fig. 4). The results showed that the GO–PVA sheets also showed reverse saturable absorption behavior at 1400 nm. The optical limiting performance at 1400 nm is poorer than that at 800 nm. Based on these nonlinear absorption coefficients obtained from the Z-scan results, and values were estimated to be 4.5 and , respectively. Furthermore, Z-scan results of the sample after exposure to 38% and 85% humidity environments for more than 24 h showed similar performance, indicating their good resistance to the moisture environment.
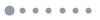
Figure 4.Open aperture Z-scan results of a GO–PVA sheet () at 1400 nm under excitation fluence of . This sample was kept in the different moisture conditions for more than 24 h before test.
It has been previously reported that nonlinear scattering plays an important role in the optical limiting properties of GO and GO–polymer composite suspensions [10,22]. Recently, we and several groups proposed that high nonlinear absorption of GO responsible for their strong optical limiting effects [18,28]. To study the mechanism responsible for the observed optical limiting of GO–PVA sheets, we have performed degenerate pump–probe measurements on the GO–PVA film with both pump and probe wavelength at 800 nm. Figure 5 shows the transient decay kinetics of GO–PVA sheets after excitation with different fluence. With low excitation fluence (), GO–PVA sheets exhibited very small photo-induced bleaching signals () at short times, which disappeared quickly within the laser pulse duration, and then the signal became negative, indicating that photo-induced absorption () occurred. However, at high excitation fluence (), the signal was completely dominated by photo-induced absorption, indicating nonlinear absorption of GO at high excitation fluence. The positive and negative pump–probe signals of GO arise from the and domains of GO sheets, respectively [28,31]. Our pump–probe results further support the nonlinear absorption behaviors of GO that was observed in fluence-dependent Z-scan measurements at high excitation fluence. The photo-induced absorption decays can be well fit with a tri-exponential decay with time constants of (30%), (45%), and (25%). It was observed that the decay time constants were independent of pump fluence. Time constants , , and are attributed to the electron–electron scattering, carrier-acoustic phonon scattering, and interband carrier recombination of quasi-fluorescent -conjugated clusters in GO sheets, respectively. The observed nonlinear absorption of GO is likely attributed to the excited-state absorption and multiphoton absorption of broad range of quasi-molecular fluorophores present in the GO sheets [28].
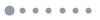
Figure 5.Excitation fluence dependent ultrafast relaxation dynamics of GO–PVA sheet () measured by femtosecond pump–probe technique. Inset shows the normalized data on a shorter time scale.
4. SUMMARY
In summary, here we report a simple method for fabrication of low-cost, flexible, highly stable, and efficient optical limiting devices by impregnating GO into PVA polymer sheets by using a solution-processing method. Such flexible GO–PVA sheets exhibited strong broadband optical limiting activity with very low optical limiting thresholds. The optical limiting thresholds are much lower than many previously reported materials. Their linear and nonlinear optical properties can be tuned by varying the amount of GO in PVA matrix. Femtosecond pump–probe measurements performed on GO–PVA sheets further support that nonlinear absorption including excited state absorption and multiphoton absorption played an important role in the observed optical limiting activities of GO–PVA sheets. Such stable and flexible optical limiting devices open doors for their practical applications as they can be attached to any optical glasses or sensitive optical detectors to protect them from intense light-induced damage. Such simple processing method could also be applied to fabrication of flexible saturable absorbers by impregnating reduced graphene or pristine graphene into polymer matrices for laser mode-locking applications.
References
[1] P. Chen, X. Wu, X. Sun, J. Lin, W. Ji, K. L. Tan. Electronic structure and optical limiting behavior of carbon nanotubes. Phys. Rev. Lett., 82, 2548-2551(1999).
[2] L. Vivien, P. Lançon, D. Riehl, F. Hache, E. Anglaret. Carbon nanotubes for optical limiting. Carbon, 40, 1789-1797(2002).
[3] L. W. Tutt, T. F. Boggess. A review of optical limiting mechanisms and devices using organics, fullerenes, semiconductors and other materials. Prog. Quantum Electron., 17, 299-338(1993).
[4] N. Venkatram, D. N. Rao, M. A. Akundi. Nonlinear absorption, scattering and optical limiting studies of CdS nanoparticles. Opt. Express, 13, 867-872(2005).
[5] L. Polavarapu, V. Mamidala, Z. P. Guan, W. Ji, Q. H. Xu. Huge enhancement of optical nonlinearities in coupled Au and Ag nanoparticles induced by conjugated polymers. Appl. Phys. Lett., 100, 023106(2012).
[6] L. Polavarapu, N. Venkatram, W. Ji, Q. H. Xu. Optical-limiting properties of oleylamine-capped gold nanoparticles for both femtosecond and nanosecond laser pulses. ACS Appl. Mater. Interfaces, 1, 2298-2303(2009).
[7] J. Wang, Y. Hernandez, M. Lotya, J. N. Coleman, W. J. Blau. Broadband nonlinear optical response of graphene dispersions. Adv. Mater., 21, 2430-2435(2009).
[8] Q. L. Bao, K. P. Loh. Graphene photonics, plasmonics, and broadband optoelectronic devices. ACS Nano, 6, 3677-3694(2012).
[9] B. Anand, A. Kaniyoor, S. S. S. Sai, R. Philip, S. Ramaprabhu. Enhanced optical limiting in functionalized hydrogen exfoliated graphene and its metal hybrids. J. Mater. Chem. C, 1, 2773-2780(2013).
[10] M. Feng, H. Zhan, Y. Chen. Nonlinear optical and optical limiting properties of graphene families. Appl. Phys. Lett., 96, 033107(2010).
[11] Z. Liu, Y. Wang, X. Zhang, Y. Xu, Y. Chen, J. Tian. Nonlinear optical properties of graphene oxide in nanosecond and picosecond regimes. Appl. Phys. Lett., 94, 021902(2009).
[12] J. Zhu, Y. Li, Y. Chen, J. Wang, B. Zhang, J. Zhang, W. J. Blau. Graphene oxide covalently functionalized with zinc phthalocyanine for broadband optical limiting. Carbon, 49, 1900-1905(2011).
[13] W. Wei, T. C. He, X. Teng, S. X. Wu, L. Ma, H. Zhang, J. Ma, Y. H. Yang, H. Y. Chen, Y. Han, H. D. Sun, L. Huang. Nanocomposites of graphene oxide and upconversion rare-earth nanocrystals with superior optical limiting performance. Small, 8, 2271-2276(2012).
[14] V. Mamidala, L. Polavarapu, J. Balapanuru, K. P. Loh, Q.-H. Xu, W. Ji. Enhanced nonlinear optical responses in donor-acceptor ionic complexes via photo induced energy transfer. Opt. Express, 18, 25928-25935(2010).
[15] G.-K. Lim, Z.-L. Chen, J. Clark, R. G. S. Goh, W.-H. Ng, H.-W. Tan, R. H. Friend, P. K. H. Ho, L.-L. Chua. Giant broadband nonlinear optical absorption response in dispersed graphene single sheets. Nat. Photonics, 5, 554-560(2011).
[16] M. B. M. Krishna, V. P. Kumar, N. Venkatramaiah, R. Venkatesan, D. N. Rao. Nonlinear optical properties of covalently linked graphene-metal porphyrin composite materials. Appl. Phys. Lett., 98, 081106(2011).
[17] Y. Gan, M. Feng, H. Zhan. Enhanced optical limiting effects of graphene materials in polyimide. Appl. Phys. Lett., 104, 171105(2014).
[18] N. Liaros, E. Koudoumas, S. Couris. Broadband near infrared optical power limiting of few layered graphene oxides. Appl. Phys. Lett., 104, 191112(2014).
[19] S. M. O’Flaherty, S. V. Hold, M. J. Cook, T. Torres, Y. Chen, M. Hanack, W. J. Blau. Molecular engineering of peripherally and axially modified phthalocyanines for optical limiting and nonlinear optics. Adv. Mater., 15, 19-32(2003).
[20] Q. L. Bao, H. Zhang, J. X. Yang, S. Wang, D. Y. Tong, R. Jose, S. Ramakrishna, C. T. Lim, K. P. Loh. Graphene–polymer nanofiber membrane for ultrafast photonics. Adv. Funct. Mater., 20, 782-791(2010).
[21] B. Zhao, B. Cao, W. Zhou, D. Li, W. Zhao. Nonlinear optical transmission of nanographene and its composites. J. Phys. Chem. C, 114, 12517-12523(2010).
[22] Z. B. Liu, Y. F. Xu, X. Y. Zhang, X. L. Zhang, Y. S. Chen, J. G. Tian. Porphyrin and fullerene covalently functionalized graphene hybrid materials with large nonlinear optical properties. J. Phys. Chem. B, 113, 9681-9686(2009).
[23] Y. S. Liu, J. Y. Zhou, X. L. Zhang, Z. B. Liu, X. J. Wan, J. G. Tian, T. Wang, Y. S. Chen. Synthesis, characterization and optical limiting property of covalently oligothiophene-functionalized graphene material. Carbon, 47, 3113-3121(2009).
[24] E. Xenogiannopoulou, K. Iliopoulos, S. Couris, T. Karakouz, A. Vaskevich, I. Rubinstein. Third-order nonlinear optical response of gold-island films. Adv. Funct. Mater., 18, 1281-1289(2008).
[25] H. Zhang, S. Virally, Q. Bao, L. Kian Ping, S. Massar, N. Godbout, P. Kockaert. Z-scan measurement of the nonlinear refractive index of graphene. Opt. Lett., 37, 1856-1858(2012).
[26] Z. Zheng, C. Zhao, S. Lu, Y. Chen, Y. Li, H. Zhang, S. Wen. Microwave and optical saturable absorption in graphene. Opt. Express, 20, 23201-23214(2012).
[27] Z. Wang, Y. Zou, Y. Chen, M. Wu, C. Zhao, H. Zhang, S. Wen. Graphene sheet stacks for Q-switching operation of an erbium-doped fiber laser. Laser Phys. Lett., 10, 075102(2013).
[28] X. F. Jiang, L. Polavarapu, S. T. Neo, T. Venkatesan, Q. H. Xu. Graphene oxides as tunable broadband nonlinear optical materials for femtosecond laser pulses. J. Phys. Chem. Lett., 3, 785-790(2012).
[29] W. S. Hummers, R. E. Offeman. Preparation of graphitic oxide. J. Am. Chem. Soc., 80, 1339(1958).
[30] A. Patra, N. Venkatram, D. N. Rao, T. P. Radhakrishnan. Optical limiting in organic molecular nano/microcrystals: nonlinear optical effects dependent on size distribution. J. Phys. Chem. C, 112, 16269-16274(2008).
[31] Z. B. Liu, X. Zhao, X. L. Zhang, X. Q. Yan, Y. P. Wu, Y. S. Chen, J. G. Tian. Ultrafast dynamics and nonlinear optical responses from sp2-and sp3-hybridized domains in graphene oxide. J. Phys. Chem. Lett., 2, 1972-1977(2011).